Understanding the mechanisms of lymphangiogenesis: a hope for cancer therapy?
1. University of Heidelberg, Medical Faculty
Mannheim, Ludolf-Krehl-Str. 13-17,
D-68167 Mannheim, Germany.
2. Forschungszentrum Karlsruhe, Institut für
Toxikologie und Genetik, Postfach 3640,
D-76021 Karlsruhe, Germany.
ABSTRACT
The last fifteen years have witnessed a rapid deepening of our understanding of both the molecular biology of lymphatic vessels and the formation of new lymphatic vessels during lymphangiogenesis. Following the discovery that VEGFR-3, a transmembrane receptor tyrosine kinase, localizes to lymphatic vessels and can orchestrate lymphangiogenesis, the list of molecular regulators of lymphangiogenesis has continued to grow, and includes growth factors, cell surface proteins, and transcription factors. In addition, molecules have been identified that are specifically expressed on lymphatic endothelial cells and therefore allow lymphangiogenesis to be monitored. These findings have in turn allowed lymphangiogenesis to be studied in a variety of diseases, most intensively in the context of cancer and metastasis. In this article I survey our current understanding of the molecular regulation of lymphangiogenesis and its relevance to metastasis and cancer patient survival. I then assess the likely efficacy of cancer therapies that target tumorassociated lymphatic vessels.
INTRODUCTION
Lymphangiogenesis describes the growth of new lymphatic vessels, usually from pre-existing lymphatic vessels in a process that is thought to be similar to angiogenesis. During this process, lymphatic endothelial cells (LECs) sprout, migrate, and proliferate in order to generate new capillaries. In addition, lymphangiogenesis includes vessel enlargement, a process that is probably driven by proliferation of LECs in the absence of sprouting and migration.
The lymphatic vasculature arises mid-gestation following establishment of the cardiovascular system (reviewed in1). Endothelial cells from the anterior cardiac vein commit to the lymphatic lineage and sprout and migrate to form the primary lymph sacs in the jugular region. Centrifugal sprouting lymphangiogenesis from these and further lymph sacs that form near other major veins, followed by merging, remodeling, and maturation of these separate lymphatic capillary networks, populates the developing embryo with its lymphatic vasculature. Mesenchymal progenitor cells may also contribute to this process.2 In the adult, lymphangiogenesis is only thought to be activated during wound healing and tissue regeneration.3-5 Pathologically, lymphangiogenesis can also be induced in chronic inflammatory lesions6,7 and in the context of tumors (see below).
A major breakthrough that has permitted lymphangiogenesis to be studied has been the discovery of proteins that are relatively specifically expressed in LECs, allowing these proteins to be used as markers of lymphatic vessels. The most important of these are the transmembrane receptor tyrosine kinase VEGFR-3, the mucin-type transmembrane glycoprotein podoplanin, the CD44-related cell surface hyaluronan receptor LYVE- 1, and the homeobox transcription factor Prox-1.8 Although these markers have been very useful, it is important to note that none of them is exclusively or homogeneously expressed on all lymphatic vessels, and therefore the detection of a combination of these markers is recommended for the reliable identification of lymphatic vessels.9
Newly formed lymphatic vessels are built from proliferating LECs. However, there is increasing evidence that bone marrow–derived and other progenitor cells may also contribute, although their relative contribution to lymphangiogenesis remains to be established. Endothelial progenitor cells, for example, have been shown to insert into existing lymphatic endothelium. Cells expressing CD34+ CD133+ VEGFR-3+ can differentiate into cells expressing vascular and lymphatic endothelial cell–specific markers.10 Consistently, bone marrow–derived cells can incorporate into the lymphatic endothelium and express lymphatic markers.11 CD11b+ LYVE-1+ macrophages have also been reported to contribute to lymphatic vessels through vascular mimicry or transdifferentiation.12,13 Furthermore, mesenchymal stem cells are able to differentiate into lymphatic endothelial cells in response to VEGF-C.14
Molecular regulation of lymphangiogenesis: from the cell surface…
The process of lymphangiogenesis is typically activated by extracellular signals such as growth factors that bind to their cognate receptor on the cell surface. As a consequence, intracellular signal transduction pathways are in turn activated that terminate in the nucleus and regulate the expression of genes responsible for orchestrating lymphangiogenesis. The archetypal molecular regulator of lymphangiogenesis on the surface of LECs is the vascular endothelial growth factor receptor family member VEGFR-3. VEGFR-3 is activated by VEGF-C and VEGF-D, members of the vascular endothelial growth factor family.15 Dimers of VEGFR-3 bind to these ligands and as a consequence tyrosine residues in the cytoplasmic portion of the dimerized receptor are trans-phosphorylated by the intracellular kinase domains of the VEGFR-3 protein. This ligandinduced autophosphorylation of VEGFR-3 activates a variety of signal transduction pathways (see below) that regulate expression of a variety of genes.16 VEGFR-3 is prominently expressed on the tip cells of sprouting lymphatic capillaries.17 These tip cells are crucial for the outgrowth of new lymphatic vessels.
VEGFR-2, another member of the vascular endothelial growth factor receptor family expressed on LECs, is also implicated in the regulation of lymphangiogenesis.18-20 The classical VEGFR-2 ligand VEGF-A can induce lymphatic hyperplasia.21,22 In addition, proteolytic cleavage of VEGF-C and VEGF-D allows these ligands to activate VEGFR-2. Activation of VEGFR-2 and VEGFR- 3 on LECs has different effects: VEGFR-2 activation leads to vessel enlargement, while VEGFR-3 activation leads to sprouting lymphangiogenesis.17 Furthermore, VEGF-D induces the formation of heterodimers between VEGFR- 2 and VEGFR-3, which may lead to differences in the signal transduction pathways that are subsequently activated.23,24 Our current understanding is that VEGFR- 2 and VEGFR-3 cooperate to regulate LEC migration and proliferation25 and that VEGFR-2 activation may be a modifier but not necessarily an initiator of lymphangiogenesis.17
A number of other cell surface molecules on LECs regulate VEGFR-2 and VEGFR-3 activity through binding to their ligands, including _9_1 integrin26 and the semaphorin co-receptor neuropilin-2.27 The _9_1 integrin binds to VEGF-A, -C and -D, while neuropilin binds to VEGF-C and -D. The lymphangiogenesisstimulating activity of VEGF-C and -D has been shown to be dependent on _9_1 integrin.26 Neuropilin-2 is cointernalized with VEGFR-3 upon ligand binding and is thought to regulate VEGFR-3 activation.27
In addition to VEGFR-2 and -3, a number of other cell surface growth factor and cytokine receptors can induce lymphangiogenesis in response to their cognate ligands. These include the receptor tyrosine kinases Tie-1 and Tie-2 and their ligands angiopoietin-1 (Ang-1) and Ang- 2,28 the hepatocyte growth factor receptor c-Met,29 EphrinB2,30 and receptors for platelet-derived growth factor,31 lymphotoxin beta,32 insulin-like growth factors 1 and 2 and members of the fibroblast growth factor family.33-35 Not all of these receptor-ligand interactions act directly to induce lymphangiogenesis. Some induce expression of pro-lymphangiogenic factors that in turn induce lymphangiogenesis, while others upregulate the expression of the receptors for these factors. Recently TGF-_ signaling has been shown to act as a negative regulator of lymphangiogenesis.36
… to the nucleus
The intracellular signal transduction pathways and transcription factors that ultimately coordinate the complex cellular processes of proliferation, migration, invasion, and tubule formation that are required for the formation of new lymphatic vessels are still being unraveled. Activation of VEGFR-3 by its ligands VEGF-C or VEGF-D results in protein kinase C–dependent activation of the MAPK signaling cascade (ERK, JNK) and induction of Akt phosphorylation.24,37 Specifically, ligandinduced phosphorylation of VEGFR-3 tyrosine residue 1063 on the cytoplasmic tail of the receptor recruits CRKI/II which in turn induces expression of the transcription factor c-jun via JNK1/2. In addition, phosphorylation of tyrosine residues 1230/1231 on the cytoplasmic portion of VEGFR-3 recruits GRB2, activating in turn ERK1/2 and AKT. Lymphangiogenic signaling by FGF-2 also activates the Akt/mTOR/p70S6 kinase pathway,38 indicating the importance of this pathway in the orchestration of lymphangiogenesis. Consistently, a specific inhibitor of mTOR called rapamycin is able to inhibit tumor-induced lymphangiogenesis and lymphatic metastasis.39 Furthermore, members of the sprouty/spred family of proteins can inhibit pro-lymphangiogenic VEGF-C signaling by suppressing VEGFR-3–mediated ERK and Akt activation.40
The cytoplasmic enzyme cyclooxygenase (COX)-2 is responsible for the synthesis of prostanoids. Recently it was reported to induce expression of VEGF-C by macrophages, and thereby to contribute to lymphangiogenesis.41 How this works at the molecular level remains to be elucidated.
One of the end points of pro-lymphangiogenic signal transduction pathways is transcriptional activation. A number of transcription factors have been implicated in determining LEC identity, including Foxc-2, Elk3 (Net), Prox1, and Sox18.15 Sox18 regulates the transcription of Prox-1,42 a homeobox transcription factor that plays a central role in determining LEC morphology and behavior43 through regulating the transcription of a battery of genes including the α9 integrin subunit.44 How these transcriptional regulators are wired into the regulatory pathways that orchestrate lymphangiogenesis largely remains unclear. In addition, the way in which transduction pathways regulate cytoskeleton dynamics and cell adhesion properties that must be central to the process of lymphangiogenesis remains to be identified. A recent insight comes from the discovery of EMS1, a secreted glycoprotein that is specifically expressed in LECs, transcriptionally upregulated by VEGF-A and -C in these cells, and which potentiates the proliferation and migration.45
Lymphangiogenesis, metastasis, and the survival of cancer patients.
More than two-thirds of all papers published about lymphangiogenesis concern cancer, making this the most intensively studied aspect of this process. Correlative studies using human tumor samples as well as functional studies in animal models provide strong evidence that tumors can induce lymphangiogenesis. It has been postulated that because of the high internal interstitial fluid pressure within tumors,46 tumor-induced lymphangiogenesis may reflect a need for increased lymphatic vessel density by tumors to drain this interstitial fluid away, although there is currently no direct evidence to support this notion. Alternatively, tumor-induced lymphangiogenesis may reflect the fact that tumors are similar to wounds that do not heal:47 mechanisms may therefore be operative in tumors that are similar to those in chronic inflammatory lesions where lymphangiogenesis is induced. Indeed, stromal cells such as tumor-associated macrophages have been implicated in the induction of tumor-induced lymphangiogenesis (see below). The idea has emerged that if tumors develop the ability to induce lymphangiogenesis, then the resulting increase in lymphatic vessel numbers in the vicinity of the tumor may consequently increase the number of tumor cells that invade the lymphatics, in turn stimulating the formation of lymph node metastases. Consistent with the fact that lymph node metastasis is a strong prognostic indicator for most carcinomas, tumor-induced lymphangiogenesis then also often correlates with poor prognosis.
The number of papers published on lymphangiogenesis and cancer has increased progressively since the year 2000, with more than 160 papers projected for 2009 (Figure 1). A detailed review of all 700 or more papers is beyond the scope of this article. I therefore summarize here the main outcomes of these studies as this research area has been reviewed in detail9,48,49 and critically evaluate the evidence that tumor-induced lymphangio – genesis contributes to lymph node metastasis and poor patient survival.
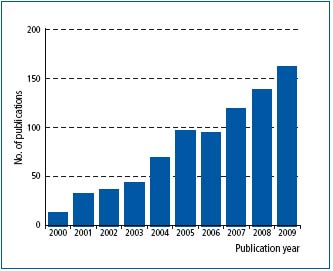
Figure 1. Graph showing the rise in publications that address lymphangiogenesis and cancer since the year 2000. Values are based on papers included in PubMed (www.pubmed.gov). The value for 2009 is projected on the basis of the number of papers published in the first quarter of 2009.
Specific markers of LECs have been used to study the location and density of lymphatic vessels in the context of tumors for a wide variety of different types of cancer. Increased numbers of lymphatic vessels have been reported peripherally within the stroma that surrounds the tumor, as well as within the tumor itself. For some but not all types of cancer, lymphatic vessel density (LVD) peritumorally and/or intratumorally has been correlated with clinical parameters such as lymph node metastasis and poor prognosis. Conflicting data have been published in the case of certain types of cancer such as breast and colorectal carcinomas, as some studies have reported a correlation, while others have not.49
In principle, tumor-associated lymphatic vessels could represent pre-existing vessels that have been co-opted by tumors, or could arise through tumor-induced lymphangiogenesis. There is evidence that both mechanisms are operative. Lymphangiogenesis would be typified by proliferation of LECs, and several studies have sought to examine the presence of proliferating LECs within tumor-associated lymphatic vessels. While some of these studies report proliferating LECs or a higher number of proliferating LECs in the tumor-associated lymphatic vessels than in lymphatic vessels from nontransformed tissue, other studies do not.49 Analysis of these data is complicated by the fact that bone marrow–derived endothelial precursor cells and CD11b+ macrophages have been reported to contribute to the lymphatic vasculature in tumors,11,50,51 although this contribution may not always be significant.52 The fact that lymphangiogenesis, vessel cooption, incorporation of progenitor cells and vascular mimicry can all contribute in principle to the lymphatic vasculature of tumors probably accounts for the lack of a tight correlation between lymphangiogenesis, LVD, lymph node metastasis, and poor prognosis. For example, if the tumor is located in an area with a high lymphatic vessel density, cooption of these vessels may obviate any need for lymphangiogenesis.
Studies of many different types of human cancers show that the expression of lymphangiogenic factors increases in more advanced malignant stages of the disease. These factors may be produced by the tumor cells themselves, or by stromal fibroblasts or tumor-associated macrophages within the tumors. The most intensively studied lymphangiogenic factors in the context of cancer are VEGF-C and -D. Many but not all such studies report a correlation between the expression of these molecules and tumor-associated LVD, lymph node metastasis, and poor prognosis.9
Tumors have been found to induce lymphangiogenesis not only locally but also distally in draining lymph nodes. In human breast tumors, lymphangiogenesis was observed in 25% of uninvolved axillary lymph nodes.53 As tumor-induced sentinel lymph node lymphangiogenesis substantially increases lymph flow to the lymph node,54 it has been postulated that lymph node metastasis may be promoted as a consequence of increased lymphatic fluid flow that brings disseminating tumor cells to tumor-draining lymph nodes.
Animal models have provided strong experimental evidence for a role for tumor-induced lymphangio – genesis in promoting metastasis. Specifically VEGF-A, VEGF-C, VEGF-D, COX-2, and PDGF-BB can contribute to tumor-induced lymphangiogenesis, as inhibition of the activity of these factors in vivo has been shown to suppress tumor-induced lymphangiogenesis (eg, 31,41,55- 57). COX-2 is likely to act indirectly by inducing expression of VEGF-C.41 The majority of these studies using animal tumor models have focused on VEGF-C and VEGF-D. Ectopic or transgenic overexpression of VEGF-C or VEGF-D in tumor cells has been shown to promote lymphangiogenesis in a variety of tumor models, as evidenced by enhanced proliferation rates in tumor-associated lymphatic vessels and increased LVD and/or lymphatic vessel diameter (reviewed in 48). Ectopic expression of these factors also concomitantly promotes metastasis in regional lymph nodes, and in many studies also in vital organs such as the lung.55,58-63 Conversely, inhibition of ligand-induced activation of VEGFR-3 in several different animal tumor models suppressed tumor-induced lymphangiogenesis but had no effect on pre-existing vessels. Importantly, this inhibition also reduced the onset or incidence of lymph node metastases, and in many cases also inhibited the formation of metastases in other organs such as the lung.55,61,64-67 These findings are consistent with the notion that VEGFR-3 activation on LECs promotes lymphangiogenesis in the tumor vicinity, thereby increasing the likelihood that invasive tumor cells will enter the lymphatic vasculature and traffic to regional lymph nodes and beyond.
Animal models also demonstrate that tumors can induce lymphangiogenesis in tumor-draining lymph nodes. Both VEGF-A and VEGF-C produced in primary tumors have been reported to do this.22,68 In some animal models, tumor-induced lymph node lymphangiogenesis has been reported in the absence of lymphangiogenesis in the vicinity of the primary tumor.54,69
In summary, there is substantial evidence that tumorinduced lymphangiogenesis does occur and is associated with metastasis, particularly within regional lymph nodes, but also in other organs. However, tumor-induced lymphangiogenesis is not an obligatory feature of tumor progression, and metastasis can occur in its absence. This reflects complex relationships between tumors and lymphatic vessels that are different not only for different types of cancer, but also for each individual tumor depending on its precise location and genetic constitution.
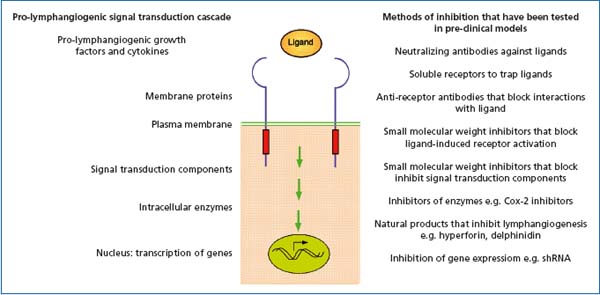
Figure 2. Signal transduction mechanisms that regulate lymphangiogenesis. Listed on the right hand side of the figure are methods for inhibiting lymphangiogenesis that target different levels of pro-lymphangiogenic signal transduction cascades.
Tumor lymphatics: targets for therapy?
The observation in animal models that inhibition of tumor-induced lymphangiogenesis is sufficient to reduce the incidence of metastasis, together with correlative studies on a variety of human cancers that connect lymphangiogenesis with poor prognosis, has raised interest in tumor lymphatics as a possible therapeutic target. For example, following the diagnosis of cancer and during subsequent therapy and remission, chronic inhibition of tumor-induced lymphangiogenesis could potentially reduce the incidence of metastasis. Another potential therapeutic setting might be in cases where patients have relatively slow-growing benign cancers, but where surgical intervention is judged to outweigh the benefit to the patient. In these cases, the tumor is often left in situ. However, a proportion of patients will progress and develop metastases (eg, 70), and thus chronic suppression of tumor-induced lymphangiogenesis may be beneficial for survival. A variety of pre-clinical models have demonstrated that lymphangiogenesis can be blocked at various levels of pro-lymphangiogenic signaling cascades, including through the use of blocking antibodies, soluble receptors, synthetic chemical inhibitors, natural substances, and shRNA (see Figure 2; reviewed in 9).
There are a number of issues to resolve regarding the therapeutic targeting of tumor-associated lymphatic vessels. As multiple factors probably contribute to tumorinduced lymphangiogenesis, blocking only one of these factors may not effectively suppress tumor-induced lymphangiogenesis. This has recently been elegantly demonstrated in animal models of pancreatic beta cell carcinoma in which tumor-induced lymphangiogenesis is driven by transgenic VEGF-C or -D expression. In these models a broad spectrum inhibitor of the VEGFR family had no effect on tumor-induced lymph – angiogenesis.71 Furthermore, as outlined above it is clear from studies on human cancers that tumor-induced lymphangiogenesis does not always contribute to the LVD in the vicinity of tumors. Inhibition of lymphangiogenesis does not seem to affect pre-existing vessels, and vessels induced in the context of chronic inflammation do not regress after withdrawal of the original pro-lymphangiogenic stimulus.7 Thus, if tumors have already induced lymphangiogenesis, or for those tumors where vessel cooption is the major source of tumor-associated lymphatics, inhibition of lymph – angiogenesis is unlikely to be effective. Possible unwanted side effects of targeting tumor-associated lymphatic vessels also have to be considered. Lymphangiogenesis is induced after wounding3 and so inhibition of lymphangiogenesis might interfere with wound healing and tissue regeneration. Other potential side effects will be dependent on the molecular pathway that is targeted.
Lymph node metastases themselves are rarely lifethreatening, despite their strong prognostic relevance.72 To have an effect on patient survival, inhibition of tumor-induced lymphangiogenesis should therefore suppress the formation not only of lymph node metastases but also metastases in vital organs. The most important question regarding the potential efficacy of targeting tumor-induced lymphangiogenesis therefore concerns the role of lymph node metastases in determining whether metastases form in other organs. As outlined above, manipulation of tumor-induced lymphangiogenesis can influence the formation of both lymph nodes metastases and metastases in vital organs such as the lung. An obvious conclusion would therefore be that lymph node metastases govern the development of metastases in other organs. This would predict that therapeutic removal of lymph nodes should have positive effects on patient survival. However, studies with large series of breast cancer and melanoma patients with follow-up often over several decades do not support this notion. In these studies, surgical removal or nonremoval of regional lymph nodes did not correlate with patient survival.73-76 Nevertheless, the development of lymph node metastases in those patients in whom regional lymph nodes were left in situ was indicative of poor prognosis. From these clinical studies, lymph node metastasis would thus seem to be an indicator but not a regulator of metastasis in vital organs.
The observation that tumor-induced lymphangiogenesis is functionally associated with metastasis in vital organs and poor prognosis needs to be reconciled with these conclusions from clinical studies. One possibility might be that pro-lymphangiogenic factors produced by tumors stimulate not only lymphangiogenesis locally, but may also have other as yet undefined systemic effects that promote metastasis. As we have seen above, prolymphangiogenic factors such as VEGF-A and -C can act systemically to induce lymphangiogenesis in regional lymph nodes. VEGF-A is also able to act systemically to induce mobilization of bone marrow–derived cells,77 which have been suggested to contribute to an organ microenvironment that is conducive to the outgrowth of disseminated tumor cells.78 In this scenario, both tumor-induced lymphangiogenesis and lymph node metastasis would serve as indicators that factors have been produced by tumors that can act systemically to promote metastasis in vital organs. However, this scenario remains a speculation.
CONCLUSIONS
The last fifteen years have witnessed the dramatic unraveling of the molecular regulation of lymphangiogenesis. In turn, this has stimulated research into the role of lymphangiogenesis in metastasis and cancer prognosis. Tumor-induced lymphangiogenesis has emerged as a mechanism that appears to contribute to metastasis and poor prognosis for cancer patients. Preclinical studies indicate that by targeting tumorinduced lymphangiogenesis it may be possible to at least partially control metastasis. However, there remain a variety of fundamental questions that need to be answered before translation of these findings into clinical applications is appropriate.
Acknowledgements: This work was supported by grants from the Deutsche Forschungsgemeinschaft under the auspices of the Schwerpunkt Program SPP1190 (Tumor-vessel interface), and from the European Union under the auspices of the FP7 collaborative project TuMIC, contract no. HEALTH-F2-2008- 201662.
REFERENCES
1. Oliver G. Lymphatic vasculature development. Nat Rev Immunol. 2004;4:35-45.
2. Wilting J, Becker J. Two endothelial cell lines derived from the somite. Anat Embryol (Berl). 2006;211 Suppl 1:57-63.
3. Paavonen K, Puolakkainen P, Jussila L, Jahkola T, Alitalo K. Vascular endothelial growth factor receptor-3 in lymphangiogenesis in wound healing. Am J Pathol. 2000;156:1499-1504.
4. Saaristo A, Tammela T, Farkkila A, et al. Vascular endothelial growth factor-C accelerates diabetic wound healing. Am J Pathol. 2006;169:1080-1087.
5. Tammela T, Saaristo A, Holopainen T, et al. Therapeutic differentiation and maturation of lymphatic vessels after lymph node dissection and transplantation. Nat Med. 2007;13:1458- 1466.
6. Pullinger D, Florey H. Proliferation of lymphatics in inflammation. J Pathol Bacteriol. 1937;45:157-170.
7. Baluk P, Tammela T, Ator E, et al. Pathogenesis of persistent lymphatic vessel hyperplasia in chronic airway inflammation. J Clin Invest. 2005;115:247-257.
8. Karpanen T, Alitalo K. Molecular biology and pathology of lymphangiogenesis. Annu Rev Pathol. 2008;3:367-397.
9. Sleeman J, Schmid A, Thiele W. Tumor lymphatics. Seminars in Cancer Biology. 2009.
10. Salven P, Mustjoki S, Alitalo R, Alitalo K, Rafii S. VEGFR-3 and CD133 identify a population of CD34+ lymphatic/vascular endothelial precursor cells. Blood. 2003;101:168- 172.
11. Jiang S, Bailey AS, Goldman DC, et al. Hematopoietic stem cells contribute to lymphatic endothelium. PLoS ONE. 2008;3:e3812.
12. Maruyama K, Ii M, Cursiefen C, et al. Inflammation-induced lymphangiogenesis in the cornea arises from CD11b-positive macrophages. J Clin Invest. 2005;115:2363-2372.
13. Kerjaschki D, Huttary N, Raab I, et al. Lymphatic endothelial progenitor cells contribute to de novo lymphangiogenesis in human renal transplants. Nat Med. 2006;12:230-234.
14. Conrad C, Niess H, Huss R, et al. Multipotent mesenchymal stem cells acquire a lymphendothelial phenotype and enhance lymphatic regeneration in vivo. Circulation. 2009;119:281-289.
15. Tammela T, Enholm B, Alitalo K, Paavonen K. The biology of vascular endothelial growth factors. Cardiovasc Res. 2005;65:550-563.
16. Eccles S, Paon L, Sleeman JP. Lymphatic metastasis: importance and new insights into cellular and molecular mechanisms. Clin Exp Metastasis. 2007;24:619-636.
17. Wirzenius M, Tammela T, Uutela M, et al. Distinct vascular endothelial growth factor signals for lymphatic vessel enlargement and sprouting. J Exp Med. 2007;204:1431-1440.
18. Jeltsch M, Kaipainen A, Joukov V, et al. Hyperplasia of lymphatic vessels in VEGF-C transgenic mice [published erratum appears in Science 1997 Jul 25;277(5325):463]. Science. 1997;276:1423-1425.
19. Veikkola T, Jussila L, Makinen T, et al. Signalling via vascular endothelial growth factor receptor-3 is sufficient for lymphangiogenesis in transgenic mice. Embo J. 2001;20:1223-1231.
20. Lohela M, Bry M, Tammela T, Alitalo K. VEGFs and receptors involved in angiogenesis versus lymphangiogenesis. Curr Opin Cell Biol. 2009.
21. Nagy JA, Vasile E, Feng D, et al. Vascular permeability factor/vascular endothelial growth factor induces lymphangiogenesis as well as angiogenesis. J Exp Med. 2002;196:1497-1506.
22. Hirakawa S, Kodama S, Kunstfeld R, Kajiya K, Brown LF, Detmar M. VEGFA induces tumor and sentinel lymph node lymphangiogenesis and promotes lymphatic metastasis. J Exp Med. 2005;201:1089-1099.
23. Dixelius J, Makinen T, Wirzenius M, et al. Ligand-induced vascular endothelial growth factor receptor-3 (VEGFR-3) heterodimerization with VEGFR-2 in primary lymphatic endothelial cells regulates tyrosine phosphorylation sites. J Biol Chem. 2003;278:40973-40979.
24. Makinen T, Veikkola T, Mustjoki S, et al. Isolated lymphatic endothelial cells transduce growth, survival and migratory signals via the VEGF-C/D receptor VEGFR-3. Embo J. 2001;20:4762-4773.
25. Goldman J, Rutkowski JM, Shields JD, et al. Cooperative and redundant roles of VEGFR-2 and VEGFR-3 signaling in adult lymphangiogenesis. Faseb J. 2007;21:1003-1012.
26. Vlahakis NE, Young BA, Atakilit A, Sheppard D. The lymphangiogenic vascular endothelial growth factors VEGF-C and -D are ligands for the integrin alpha9beta1. J Biol Chem. 2005;280:4544-4552.
27. Karpanen T, Heckman CA, Keskitalo S, et al. Functional interaction of VEGF-C and VEGF-D with neuropilin receptors. Faseb J. 2006;20:1462-1472.
28. Gale NW, Thurston G, Hackett SF, et al. Angiopoietin-2 is required for postnatal angiogenesis and lymphatic patterning, and only the latter role is rescued by Angiopoietin-1. Dev Cell. 2002;3:411-423.
29. Kajiya K, Hirakawa S, Ma B, Drinnenberg I, Detmar M. Hepatocyte growth factor promotes lymphatic vessel formation and function. Embo J. 2005;24:2885-28895.
30. Makinen T, Adams RH, Bailey J, et al. PDZ interaction site in ephrinB2 is required for the remodeling of lymphatic vasculature. Genes Dev. 2005;19:397-410.
31. Cao R, Bjorndahl MA, Religa P, et al. PDGF-BB induces intratumoral lymphangiogenesis and promotes lymphatic metastasis. Cancer Cell. 2004;6:333-345.
32. Furtado GC, Marinkovic T, Martin AP, et al. Lymphotoxin beta receptor signaling is required for inflammatory lymphangiogenesis in the thyroid. Proc Natl Acad Sci U S A. 2007;104:5026-5031.
33. Chang LK, Garcia-Cardena G, Farnebo F, et al. Dose-dependent response of FGF-2 for lymphangiogenesis. Proc Natl Acad Sci U S A. 2004;101:11658-11663.
34. Shin JW, Min M, Larrieu-Lahargue F, et al. Prox1 promotes lineage-specific expression of fibroblast growth factor (FGF) receptor-3 in lymphatic endothelium: a role for FGF signaling in lymphangiogenesis. Mol Biol Cell. 2006;17:576-584.
35. Achen MG, Stacker SA. Tumor lymphangiogenesis and metastatic spread-new players begin to emerge. Int J Cancer. 2006;119:1755-1760.
36. Oka M, Iwata C, Suzuki HI, et al. Inhibition of endogenous TGF-beta signaling enhances lymphangiogenesis. Blood. 2008;111:4571-4579.
37. Salameh A, Galvagni F, Bardelli M, Bussolino F, Oliviero S. Direct recruitment of CRK and GRB2 to VEGFR-3 induces proliferation, migration, and survival of endothelial cells through the activation of ERK, AKT, and JNK pathways. Blood. 2005;106:3423-3431.
38. Matsuo M, Yamada S, Koizumi K, Sakurai H, Saiki I. Tumour-derived fibroblast growth factor-2 exerts lymphangiogenic effects through Akt/mTOR/p70S6kinase pathway in rat lymphatic endothelial cells. Eur J Cancer. 2007;43:1748-1754.
39. Kobayashi S, Kishimoto T, Kamata S, Otsuka M, Miyazaki M, Ishikura H. Rapamycin, a specific inhibitor of the mammalian target of rapamycin, suppresses lymphangiogenesis and lymphatic metastasis. Cancer Sci. 2007;98:726-733.
40. Taniguchi K, Kohno R, Ayada T, et al. Spreds are essential for embryonic lymphangiogenesis by regulating vascular endothelial growth factor receptor 3 signaling. Mol Cell Biol. 2007;27:4541-4550.
41. Iwata C, Kano MR, Komuro A, et al. Inhibition of cyclooxygenase-2 suppresses lymph node metastasis via reduction of lymphangiogenesis. Cancer Res. 2007;67:10181-89.
42. Francois M, Caprini A, Hosking B, et al. Sox18 induces development of the lymphatic vasculature in mice. Nature. 2008;456:643-647.
43. Johnson NC, Dillard ME, Baluk P, et al. Lymphatic endothelial cell identity is reversible and its maintenance requires Prox1 activity. Genes Dev. 2008;22:3282-3291.
44. Mishima K, Watabe T, Saito A, et al. Prox1 induces lymphatic endothelial differentiation via integrin alpha9 and other signaling cascades. Mol Biol Cell. 2007;18:1421-1429.
45. Shin JW, Huggenberger R, Detmar M. Transcriptional profiling of VEGF-A and VEGF-C target genes in lymphatic endothelium reveals endothelialspecific molecule-1 as a novel mediator of lymphangiogenesis. Blood. 2008;112:2318-2326.
46. Jain RK. Delivery of novel therapeutic agents in tumors: physiological barriers and strategies. J Natl Cancer Inst. 1989;81:570-576.
47. Dvorak HF. Tumors: wounds that do not heal. Similarities between tumor stroma generation and wound healing. N Engl J Med. 1986;315:1650-1659.
48. Thiele W, Sleeman JP. Tumor-induced lymphangiogenesis: A target for cancer therapy? J Biotechnol. 2006;124:224- 241.
49. Sleeman J, Thiele W. Tumor metastasis and the lymphatic vasculature. Int J Cancer. 2009;125:2747-2756.
50. Schledzewski K, Falkowski M, Moldenhauer G, et al. Lymphatic endothelium-specific hyaluronan receptor LYVE-1 is expressed by stabilin-1+, F4/80+, CD11b+ macrophages in malignant tumours and wound healing tissue in vivo and in bone marrow cultures in vitro: implications for the assessment of lymphangiogenesis. J Pathol. 2006;209:67-77.
51. Religa P, Cao R, Bjorndahl M, Zhou Z, Zhu Z, Cao Y. Presence of bone marrow-derived circulating progenitor endothelial cells in the newly formed lymphatic vessels. Blood. 2005;106:4184-4190.
52. He Y, Rajantie I, Ilmonen M, et al. Preexisting lymphatic endothelium but not endothelial progenitor cells are essential for tumor lymphangiogenesis and lymphatic metastasis. Cancer Res. 2004;64:3737-3740.
53. Van den Eynden GG, Van der Auwera I, Van Laere SJ, et al. Induction of lymphangiogenesis in and around axillary lymph node metastases of patients with breast cancer. Br J Cancer. 2006;95:1362-1366.
54. Harrell MI, Iritani BM, Ruddell A. Tumor-induced sentinel lymph node lymphangiogenesis and increased lymph flow precede melanoma metastasis. Am J Pathol. 2007;170:774- 786.
55. Krishnan J, Kirkin V, Steffen A, et al. Differential in vivo and in vitro expression of vascular endothelial growth factor (VEGF)-C and VEGF-D in tumors and its relationship to lymphatic metastasis in immunocompetent rats. Cancer Res. 2003;63:713-722.
56. Shibata MA, Morimoto J, Shibata E, Otsuki Y. Combination therapy with short interfering RNA vectors against VEGF-C and VEGF-A suppresses lymph node and lung metastasis in a mouse immunocompetent mammary cancer model. Cancer Gene Ther. 2008;15:776-786.
57. Da MX, Wu Z, Tian HW. Tumor lymphangiogenesis and lymphangiogenic growth factors. Arch Med Res. 2008;39:365-372.
58. Mandriota SJ, Jussila L, Jeltsch M, et al. Vascular endothelial growth factor- C-mediated lymphangiogenesis promotes tumour metastasis. Embo J. 2001;20:672-682.
59. Skobe M, Hawighorst T, Jackson DG, et al. Induction of tumor lymphangiogenesis by VEGF-C promotes breast cancer metastasis. Nat Med. 2001;7:192-198.
60. Stacker SA, Caesar C, Baldwin ME, et al. VEGF-D promotes the metastatic spread of tumor cells via the lymphatics. Nat Med. 2001;7:186-191.
61. He Y, Kozaki K, Karpanen T, et al. Suppression of tumor lymphangiogenesis and lymph node metastasis by blocking vascular endothelial growth factor receptor 3 signaling. J Natl Cancer Inst. 2002;94:819-825.
62. Hirakawa S, Brown LF, Kodama S, Paavonen K, Alitalo K, Detmar M. VEGF-C-induced lymphangiogenesis in sentinel lymph nodes promotes tumor metastasis to distant sites. Blood. 2007;109:1010-1017.
63. Kopfstein L, Veikkola T, Djonov VG, et al. Distinct roles of vascular endothelial growth factor-D in lymphangiogenesis and metastasis. Am J Pathol. 2007;170:1348-1361.
64. He XW, Liu T, Chen YX, et al. Calcium carbonate nanoparticle delivering vascular endothelial growth factor-C siRNA effectively inhibits lymphangiogenesis and growth of gastric cancer in vivo. Cancer Gene Ther. 2008;15:193-202.
65. Thelen A, Scholz A, Benckert C, et al. VEGF-D promotes tumor growth and lymphatic spread in a mouse model of hepatocellular carcinoma. Int J Cancer. 2008;122:2471-2481.
66. Matsui J, Funahashi Y, Uenaka T, Watanabe T, Tsuruoka A, Asada M. Multi-kinase inhibitor E7080 suppresses lymph node and lung metastases of human mammary breast tumor MDA-MB-231 via inhibition of vascular endothelial growth factorreceptor (VEGF-R) 2 and VEGF-R3 kinase. Clin Cancer Res. 2008;14:5459- 5465.
67. Burton JB, Priceman SJ, Sung JL, et al. Suppression of prostate cancer nodal and systemic metastasis by blockade of the lymphangiogenic axis. Cancer Res. 2008;68:7828-7837.
68. Hirakawa S, Kodama S, Kunstfeld R, Kajiya K, Brown LF, Detmar M. VEGFA induces tumor and sentinel lymph node lymphangiogenesis and promotes lymphatic metastasis. J Exp Med. 2005;201:1089-1099.
69. Ruddell A, Kelly-Spratt KS, Furuya M, Parghi SS, Kemp CJ. p19/Arf and p53 suppress sentinel lymph node lymphangiogenesis and carcinoma metastasis. Oncogene. 2008;27:3145- 3155.
70. Matzkin H, Patel JP, Altwein JE, Soloway MS. Stage T1A carcinoma of prostate. Urology. 1994;43:11-21.
71. Schomber T, Zumsteg A, Strittmatter K, et al. Differential effects of the vascular endothelial growth factor receptor inhibitor PTK787/ZK222584 on tumor angiogenesis and tumor lymphangiogenesis. Mol Cancer Ther. 2009;8:55-63.
72. Cady B. Regional lymph node metastases; a singular manifestation of the process of clinical metastases in cancer: contemporary animal research and clinical reports suggest unifying concepts. Ann Surg Oncol. 2007;14:1790-1800.
73. Fisher B, Redmond C, Fisher ER. The contribution of recent NSABP clinical trials of primary breast cancer therapy to an understanding of tumor biology—an overview of findings. Cancer. 1980;46:1009-1025.
74. Veronesi U, Orecchia R, Zurrida S, et al. Avoiding axillary dissection in breast cancer surgery: a randomized trial to assess the role of axillary radiotherapy. Ann Oncol. 2005;16:383- 388.
75. Veronesi U, Marubini E, Mariani L, Valagussa P, Zucali R. The dissection of internal mammary nodes does not improve the survival of breast cancer patients. 30-year results of a randomised trial. Eur J Cancer. 1999;35:1320-1325.
76. Pharis DB, Zitelli JA. The management of regional lymph nodes in cancer. Br J Dermatol. 2003;149:919-925.
77. Pitchford SC, Furze RC, Jones CP, Wengner AM, Rankin SM. Differential mobilization of subsets of progenitor cells from the bone marrow. Cell Stem Cell. 2009;4:62-72.
78. Kaplan RN, Riba RD, Zacharoulis S, et al. VEGFR1-positive haematopoietic bone marrow progenitors initiate the pre-metastatic niche. Nature. 2005;438:820-827.