Long-haul travel venous thromboembolism – an update
Alun H. DAVIE
Department of Surgery and Cancer,
Imperial College
Abstract
Venous thromboembolism (VTE) describes obstructive presentations of deep venous disease, including deep vein thrombosis and pulmonary embolism, which, together, affect up to half a million people in Europe every year. VTE is also the single, most common cause of preventable hospital-acquired mortality; furthermore, up to 50% of individuals with deep vein thrombosis develop the postthrombotic syndrome, with its significant impact on quality of life and a considerable economic burden. Immobility is amongst the most important recognized risk factors for VTE. Long-haul travel, where individuals are in a forced state of relative immobility for prolonged periods, has been associated with VTE development, notably in the context of the “economy class syndrome.” However, the effect of the type and duration of travel, as well as the mechanisms through which long-haul travel increases VTE risk, are poorly characterized. This narrative review aims to summarize the existing evidence on long-haul travel and VTE, with considerations on the pathophysiology underlying the development of thrombotic events, factors increasing risk and what conservative and pharmacological measures can be taken to reduce VTE risk, particularly in the context of planned intervention.
Epidemiology and disease burden
Venous thromboembolism (VTE) is a leading cause of morbidity and mortality worldwide, and it is the single most common cause of hospital-acquired mortality. It is estimated that 10 million cases of VTE occur every year worldwide, although this may be an underestimation of the true prevalence due to the lack of reported VTE events and the presence of subclinical VTEs in patients with sudden deaths.1
VTE describes the presence of thrombosis in the deep venous system; this includes deep venous thrombosis (DVT), which, in 30% of individuals, can progress to the development of a pulmonary embolism. Postthrombotic syndrome (PTS) develops in 50% of patients with DVT and is characterized by chronic signs and symptoms of venous insufficiency, including venous claudication, swelling and, in the most severe cases, venous leg ulceration.
VTE is responsible for an estimated $27.2 billion expenditure in the US per annum between actual and preventable costs.2 This expenditure is due not only to the costs of the acute management of VTE and ongoing care for PTS, but also secondary to the lost productivity due to loss of work days, resulting in societal costs. Due to its significant morbidity and mortality, VTE prevention is key, which is performed by providing either pharmacological or mechanical thromboprophylaxis to individuals deemed at risk of developing this condition.
Risk factors
Risk factors for VTE are numerous and can be both innate and acquired. Innate factors include age, male sex (especially in the older population), ethnicity, and the presence of an underlying thrombophilia. Acquired factors include a diagnosis of cancer, the presence of significant comorbidities, a family history of VTE, varicose veins, recent pregnancy, undergoing surgery with immobility, trauma or pelvic/long bone fractures, a critical care admission, obesity, and medications, such as hormone replacement therapy or the oral contraceptive pill.3
The relative importance, or weight, of different VTE risk factors has been explored, leading to the development of risk assessment tools that permit assessment of patients based on their risk factors and their risk stratification, guiding VTE prophylaxis. In the UK, the Department of Health risk assessment tool is most commonly used,4 while, in the US, the Caprini risk assessment tool is often used, particularly in surgical patients5; the Padua risk assessment tool is used in medical patients.6 Immobility features in these risk scoring systems as an important risk factor. The Department of Health tool mentions “significantly reduced mobility for 3 days or more” and “surgery with significant reduction in mobility6”; the Caprini risk assessment tool includes “on bed rest or restricted mobility, including a removable leg brace, for less than 72 hours” (1 point additional risk), and “confined to bed for 72 hours or more” (2 point additional risk),5 while the Padua risk assessment tool mentions “reduced mobility.6” However, no tool specifically mentions long-haul travel as an independent risk factor, despite its known association with VTE. This is an important consideration as there is evidence that factors other than immobility can lead to a prothrombotic state, which may be of relevance for patients being admitted to the hospital.
Long-haul travel and VTE
Air travel is now one of the main modes of transportation, with direct flights available for increasingly longer routes. The longest existing flight is the Singapore Airlines service from Singapore to Newark, New Jersey, which has a duration of 19 hours.7 Long-haul travel is normally defined by flight length greater than 6 hours, although the definition can be variable in the literature; this has also been defined as flight length greater than 4 hours (Table I).
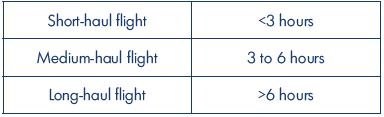
Table I. Flight length definitions.
From reference 8: McGinley Group. The differences between
long-haul & short-haul piloting. https://www.mcginleygroup.
co.uk/blog/the-differences-between-longhaul-and-shorthaulpiloting/
bp67. Published July 2015.
The 2018 International Air Transport Association (IATA) forecast predicts that, by 2037, air passenger numbers could double to 8.2 billion, which will be associated with a shift in travel toward the Asia-Pacific region, which will drive the largest growth of new passengers (Figure 1),9 and will likely result in a larger number of individuals travelling on long-haul flights.
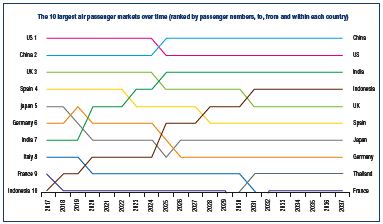
Figure 1. Predicted growth in the 10 largest air passenger
markets in the next 20 years.
From reference 9: International Air Transport Association. IATA
forecast predicts 8.2 billion air travelers in 2037. Published
October 2018.
The first report associating long-haul travel with VTE was by John Homans in 1954, who described the development of a DVT in one of his colleagues travelling from Boston to Caracas on an 8-hour flight.10 Since then, the relationship between long-haul travel and VTE has been highlighted and often described by the term “economy class syndrome.” Numerous airlines have taken measures to reduce this risk by providing passenger advice on how to recognize risk factors for VTE and suggestions for calf muscle pump exercises aimed at reducing the risk of thrombus formation, though the evidence for this is sparse.11 The World Health Organization research into global hazards of travel (WHO WRIGHT) project highlighted the research priority of setting up multicenter, international epidemiological, prospective studies to obtain more robust data on the incidence and prevalence of travel-related VTE,12 with the aim of improving the existing evidence on the subject.
Estimation of travel-related VTE prevalence is challenging for a number of reasons because there is a lack of evidence in the literature, with a limited number of studies that are heterogeneous in nature and with limited patient numbers. Furthermore, although patients with symptomatic VTE may be identified when presenting to health care professionals for assessment, the proportion of subclinical VTE events is likely much higher. It is important to note that prospective study design in this patient population is challenging, requiring duplex ultrasound screening of large numbers of participants undertaking long-haul flights.
Retrospective data has estimated the overall incidence of pulmonary embolism as 0.39 per one million passengers; for flights up to 8 hours, the incidence was 0.25, while, for flights longer than 8 hours, this increased to 1.65 per one million passengers.13 A meta-analysis exploring the relationship between travel >8 hours and VTE events revealed a relative risk for VTE of 2.8, with an 18% additional risk for each 2-hour increase in travel duration by any mode; interestingly, the increased relative risk for air travel was 26% for every 2 additional hours, suggesting that flying conferred an increased risk of VTE compared with other forms of transportation.14
The mechanism for the development of travel-related VTE has been the subject of a number of research studies. Candidate mechanisms include hypobaric hypoxia, immobilization, and stress.15,16
Air travel is associated with a hypoxic environment in the context of decreased cabin pressure, which is equivalent to approximately what is found at an altitude of 1524 to 2134 meters. Associated with this is a reduced oxygen partial pressure, with resulting saturations of approximately 93% in healthy individuals, although people with underlying cardiac or respiratory conditions may be affected more severely.17 Studies have replicated these conditions experimentally, for example, by sampling participants in Antarctica,18 where hypobaric hypoxia is present due to atmospheric conditions, or by employing hypobaric chambers,19 with the aim of investigating the role of hypobaric hypoxia in the development of a procoagulant state. These studies have provided evidence that markers of coagulation activation, fibrinolysis, and platelet and endothelial cell activation do not significantly vary between hypobaric and normobaric experimental exposures,19 including D-dimer, thrombin antithrombin complexes, and prothrombin fragments.18 It is important to note that patients with a previous history of VTE,18,19 or testing positive for Factor V Leiden or prothrombin mutations were excluded from these studies,19 with the aim of isolating the true effect of the experimental conditions on the development of thrombosis.
Conversely, a separate study assessing patients following an 8-hour flight found an increase in prothrombin fragments, thrombin antithrombin complex, and D-dimer; these increases were particularly marked in individuals with a Factor V Leiden mutation who also took oral contraceptives, suggesting that additional factors may increase travel related VTE risk in addition to hypobaric hypoxia.20
Immobilization is one of the most important risk factors for VTE, as evidenced by its inclusion in the risk assessment tools. However, the evidence for this, in the context of travel thrombosis, is unclear, with some studies suggesting that prolonged immobilization does not affect hypercoagulability,18 while others are demonstrating increased prothrombotic effects. In a study investigating healthy women who underwent 60 days of bed rest, there was no evidence of an activated coagulation system.18
Individuals exposed to two sessions of immobilization lasting 8 hours (8-hour flight, 8-hour movie marathon) and one session of regular daily activities lasting 8 hours (each activity separated by at least 2 weeks) did not demonstrate statistically significant increases in procoagulant factors in the blood during immobilization, although the use of the oral contraceptive pill and the presence of a Factor V Leiden mutation increased the levels, particularly after a flight. Therefore, it is likely that immobilization alone is not enough to confer an increased VTE risk in healthy participants, unless other risk factors are present.20 Despite being typically associated with VTE development, there is no evidence that travelling in economy class confers a higher risk,21 suggesting that the “economy class syndrome” may be a misnomer.
One of the main drawbacks in the presented literature is that study sizes are limited, ranging from 24 to 73 participants.18-20 Larger studies have explored other risk factors of relevance in individuals who have developed VTE in the context of long-haul travel.
Additional VTE risk factors appear to play an important role in determining who is likely to have an event. In a large study enrolling 568 patients, individuals who had a proven DVT did not have a statistically significant association with a history of air travel >8 hours. However, the presence of any additional single VTE risk factor conferred a statistically significant increase in the odds of developing VTE for travel >3 hours and >8 hours, suggesting that the cumulative effect of increased risk factors in addition to the length of flight can influence the development of a clinically symptomatic VTE.22 Risk factors included hormonal therapy, surgery, malignancy, immobilization, pregnancy, obesity, previous thrombosis, and significant family history. A further questionnaire-based study identified a dose-dependent effect of flying time on VTE risk, with flights >12 hours associated with an odds ratio (OR) of 2.75 for VTE development, while those of 4 to 8 hours had an OR of 1.81. Interestingly, previous surgery within 28 days conferred the highest increase in risk, with an overall OR >30; when sub-grouped by low- (day case or overnight stay), moderate-, and high-risk surgery, OR values were 5.4, 36.6, and 141.7, respectively. Previous VTE (OR, 9) and obesity (OR, 2.7) were other risk factors of importance in increasing VTE risk.23 The importance of concurrent risk factors in increasing the risk of flight-related VTE was also highlighted by the LONFLIT 1 study (LONg-haul FLIghTs deep vein thrombosis), where only the high-risk VTE group developed thrombotic events (2.8% versus 0% in the lowrisk VTE group).24 A further study identified increased risk in those with repeated flight exposures, with increasing flight duration, in those aged <30, women on oral contraceptives, and participants who were short (<1.65 m), tall (>1.8 m), or overweight (>25 kg/m2).25
There is evidence that the increase in VTE risk following a long-haul flight can last for a number of weeks. A study recruiting 8755 participants exposed to flights of at least 4 hours or more (defined as long-haul flights) recorded a VTE incidence of 1 event per 4656 long-haul flights, of which 42% occurred in the first 8 weeks following travel, with particularly high rates in the first 2 weeks.25
The MEGA study is among the largest cohorts investigating the relationship between VTE and long-haul travel (defined as >4 hours), recruiting 1906 patients with a first episode of venous thrombosis.26 This study found that travelling increased the odds of developing VTE two-fold, regardless of the mode, with travel by car, bus, or train resulting in a similar risk. Patients with known hypercoagulability, such as Factor V Leiden mutation, obesity, and on the oral contraceptive pill, were at a particularly high risk of developing VTE when traveling by car, bus, or train. Interestingly, an association with height greater than 1.90 meters also conferred an OR of 5 for the development of VTE with any form of travel, while those shorter than 1.60 meters had a 5-fold increase in the risk of developing VTE following air travel. The risk of VTE was highest in the first week, but remained elevated up to 6 weeks posttravel.
In travelers with symptomatic VTEs following international flights from Australia, the reported risk of VTE was 4-fold 2 to 4 weeks following travel, suggesting that the prothrombotic state is maintained.27
The aforementioned studies have suggested a relationship, which is likely multifactorial and associated with the presence of a number of risk factors, between VTE and long-haul travel. With respect to measures that can be taken to preemptively reduce VTE risk, the LONFLIT studies explored this with duplex ultrasound performed 24 hours following a long-haul flight. LONFLIT 2 evaluated high risk individuals for VTE; risk factors included a previous history of DVT, reduced mobility, neoplastic disease within the previous 2 years, severe obesity, large varicose veins, and a documented coagulation disorder. Participants were randomized to no thromboprophylaxis (n=422) and mechanical thromboprophylaxis via below-knee compression stockings (n=411, class I or class II). The control group had a VTE prevalence of 4.5%, while the compression stocking group had a prevalence of 0.24%, demonstrating an 18-fold difference.24 The effectiveness of graduated compression stockings in preventing travel-related VTE has been highlighted by a recent Cochrane review, which evidenced a large reduction is asymptomatic DVT with high-quality evidence and a reduction in leg swelling with low-quality evidence. There was also moderate-quality evidence suggesting that superficial vein thrombosis events were reduced in the compression group. The review could not comment on symptomatic DVT, pulmonary embolism, or death due to the paucity of these events occurring in the trials included.28
LONFLIT 3 explored the use of aspirin versus low-molecular weight heparin (LMWH) in 300 high-risk patients who were randomized to no thromboprophylaxis, 400 mg of aspirin (duration of 3 days starting 12 hours before the flight), or LMWH (single dose of 1000 IU per body weight delivered 2 to 4 hours before the flight).29 A substantial proportion of the study population (17%) was lost to follow up; nonetheless, thrombotic events for the three groups (including superficial and deep vein thrombosis) were 4.8% in the control arm, 3.6% in the aspirin arm, and 0.6% in the LMWH arm. The authors suggested that LMWH dosing as a single intervention prior to a long-haul flight in high-risk patients should be considered. Interestingly, the vast majority of thrombotic events, 85%, were observed in nonaisle seats, suggesting that reduced mobility associated with a seated position may be a contributing factor to the development of thrombotic episodes.
Guidance and relevance to superficial venous intervention
The American College of Chest Physicians Clinical Practice Guidelines for the prevention of VTE in nonsurgical patients quote a relative risk of VTE of 2.8 with long-haul travel; risk factors associated included immobility, sitting in a window seat, obesity, and recent surgery. They advise, with a grade 2C recommendation, frequent ambulation, calf muscle exercises, and sitting in an aisle seat for high-risk, long-haul travelers.30 Graduated compression stockings delivering pressures of 15 to 30 mm Hg are also recommended with a grade 2C recommendation for high risk patients, though not for any other travelers. The routine use of pharmacological thromboprophylaxis in all long-haul travelers is not recommended (grade C).
The National Health Service (NHS) website provides advice relating to when patients can fly following an intervention.31 The range is from 1 to 2 days after keyhole surgery to 10 days following coronary artery bypass grafting or more complicated abdominal surgery. There is no specific information regarding long-haul flights or flying following superficial venous intervention. Minimally invasive superficial venous interventions carry a risk of VTE that is less than 1%32; however, there is an association between the presence of varicose veins and the development of VTE, as described in international risk assessment tools.5 Therefore, this population may be at an increased risk of developing flight-related VTE events. Formal guidance, however, is not available.
Based on the existing evidence, the risk with a short-haul flight may not be significant. For medium- to long-haul flights (4 to 6 hours), however, it may be advisable to delay a superficial venous intervention for at least 2 weeks following air travel, due to reported increased VTE rates.
There is no evidence to provide advice on the timing of travel following superficial venous intervention. Advice should be based on an individual patient’s risk factor assessment, bearing in mind travel duration, with consideration of additional mechanical and/or chemical thromboprophylactic measures. Currently, there is no accepted standard regarding routine thromboprophylaxis in the context of superficial venous interventions, although individual units use thromboprophylaxis.33,34
Conclusion
This review highlights that VTE prevalence is increased during long-haul travel, but that the increased risk is likely due to a combination of factors, as opposed to travel alone. The risk of VTE is reported to be highest in the first 2 weeks following travel, which must be taken into account when planning an intervention. There is evidence that compression stockings reduce VTE rates in travelers, and targeted pharmacological thromboprophylaxis may be suitable in select high-risk patients.
Further studies are required to clarify the relationship between long-haul travel and VTE in the context of patients with underlying venous disease.
REFERENCES
1. White RH. The epidemiology of venous thromboembolism. Circulation. 2003;107(23 suppl 1):I4-I8.
2. Mahan CE, Borrego ME, Woersching AL, et al. Venous thromboembolism: annualised United States models for total, hospital-acquired and preventable costs utilising long-term attack rates. Thromb Haemost. 2012;108(2):291-302.
3. Anderson FA Jr, Spencer FA. Risk factors for venous thromboembolism. Circulation. 2003;107(23 suppl 1):I9-I16.
4. National Institute for Health and Clinical Excellence. Department of Health Risk Assessment Tool for Venous Thromboembolism (VTE). https://www. nice.org.uk/guidance/ng89/resources/ department-of-health-vte-risk-assessmenttool- pdf-4787149213. Published 2010. Accessed August 2019.
5. Illinois State Medical Society. https:// www.venousdisease.com/caprini-dvt-riskassessment. pdf. Published February 2013. Accessed June 27, 2017.
6. Barbar S, Noventa F, Rossetto V, et al. A risk assessment model for the identification of hospitalized medical patients at risk for venous thromboembolism: the Padua Prediction Score. J Thromb Haemost. 2010;8:2450- 2457.
7. Kellner T. Now boarding the world’s longest flight: a passenger jet from singapore lands in Newark after nearly 18 hours. https://www.ge.com/reports/ now-boarding-worlds-longest-flightsingapore- newark-takes-nearly-18-hours. Published October 2018. Accessed August 2019.
8. McGinley Group. The differences between long-haul & short-haul piloting. https://www.mcginleygroup.co.uk/blog/ the-differences-between-longhaul-andshorthaul- piloting/bp67. Published July 2015. Accessed August 2019.
9. International Air Transport Association. IATA forecast predicts 8.2 billion air travelers in 2037. Published October 2018. Accessed August 2019.
10. Homans J. Thrombosis of the deep leg veins due to prolonged sitting. N Engl J Med. 1954;250(4):148-149.
11. Scurr JR, Ahmad N, Thavarajan D, Fisher RK. Traveller’s thrombosis: airlines still not giving passengers the WRIGHT advice. Phlebology. 2010;25(5):257-260.
12. World Health Organization. WHO research into global hazards of travel (WrIGHt) project. https://www.who.int/ cardiovascular_diseases/wright_project/ phase1_report/en/. Published 2007. Accessed August 2019.
13. Pérez-Rodríguez E, Jiménez D, Díaz G, et al. Incidence of air travel-related pulmonary embolism at the Madrid- Barajas airport. Arch Intern Med. 2003;163(22):2766-2770.
14. Chandra D, Parisini E, Mozaffarian D. Meta-analysis: travel and risk for venous thromboembolism. Ann Intern Med. 2009;151(3):180-190.
15. Symington IS, Stack BH. Pulmonary thromboembolism after travel. Br J Dis Chest. 1977;71(2):138-140.
16. Sándor T. Travel thrombosis: pathomechanisms and clinical aspects. Pathophysiology. 2008;15(4):243-252.
17. Mohr LC. Hypoxia during air travel in adults with pulmonary disease. Am J Med Sci. 2008;335(1):71-79.
18. Venemans-Jellema A, Schreijer AJ, Le Cessie S, Emmerich J, Rosendaal FR, Cannegieter SC. No effect of isolated long-term supine immobilization or profound prolonged hypoxia on blood coagulation. J Thromb Haemost. 2014;12(6):902-909.
19. Toff WD, Jones CI, Ford I, et al. Effect of hypobaric hypoxia, simulating conditions during long-haul air travel, on coagulation, fibrinolysis, platelet function, and endothelial activation. JAMA. 2006;295(19):2251-2261.
20. Schreijer AJ, Cannegieter SC, Meijers JC, Middeldorp S, Büller HR, Rosendaal FR. Activation of coagulation system during air travel: a crossover study. Lancet. 2006;367(9513):832-838.
21. Jacobson BF, Münster M, Smith A, et al. The BEST study–a prospective study to compare business class versus economy class air travel as a cause of thrombosis. S Afr Med J. 2003;93(7):522-528.
22. Arya R, Barnes JA, Hossain U, Patel RK, Cohen AT. Long-haul flights and deep vein thrombosis: a significant risk only when additional factors are also present. Br J Haematol. 2002;116(3):653-654.
23. MacCallum PK, Ashby D, Hennessy EM, et al. Cumulative flying time and risk of venous thromboembolism. Br J Haematol. 2011;155(5):613-619.
24. Belcaro G, Geroulakos G, Nicolaides AN, MyersKA, Winford M. Venous thromboembolism from air travel: the LONFLIT study. Angiology. 2001;52(6):369-374.
25. Kuipers S, Cannegieter SC, Middeldorp S, Robyn L, Büller HR, Rosendaal FR. The absolute risk of venous thrombosis after air travel: a cohort study of 8,755 employees of international organisations. PLoS Med. 2007;4(9):e290.
26. Cannegieter SC, Doggen CJ, van Houwelingen HC, Rosendaal FR. Travelrelated venous thrombosis: results from a large population-based case control study (MEGA study). PLoS Med. 2006;3(8):e307.
27. Kelman CW, Kortt MA, Becker NG, et al. Deep vein thrombosis and air travel: record linkage study. BMJ. 2003;327(7423):1072.
28. Clarke MJ, Broderick C, Hopewell S, Juszczak E, Eisinga A. Compression stockings for preventing deep vein thrombosis in airline passengers. Cochrane Database Syst Rev. 2016;9:CD004002.
29. Cesarone MR, Belcaro G, Nicolaides AN, et al. Venous thrombosis from air travel: the LONFLIT3 study–prevention with aspirin vs low-molecular-weight heparin (LMWH) in high-risk subjects: a randomized trial. Angiology. 2002;53(1):1-6.
30. Kahn SR, Lim W, Dunn AS, et al. Prevention of VTE in nonsurgical patients: Antithrombotic Therapy and Prevention of Thrombosis, 9th ed: American College of Chest Physicians Evidence-Based Clinical Practice Guidelines. Chest. 2012;141(suppl 2):e195S-e226S.
31. National Health Service. When can I fly after surgery? https://www.nhs.uk/ common-health-questions/accidentsfirst- aid-and-treatments/when-can-i-flyafter- surgery. Published October 2018. Accessed August 2019.
32. Brittenden J, Cotton SC, Elders A, et al. Clinical effectiveness and costeffectiveness of foam sclerotherapy, endovenous laser ablation and surgery for varicose veins: results from the Comparison of LAser, Surgery and foam Sclerotherapy (CLASS) randomised controlled trial. Health Technol Assess. 2015;19(27):1-342.
33. Lane T, Bootun R, Dharmarajah B et al. A multi-centre randomised controlled trial comparing radiofrequency and mechanical occlusion chemically assisted ablation of varicose veins – final results of the Venefit versus Clarivein for varicose veins trial. Phlebology. 2017;32(2):89-98.
34. Keo HH, Baumann F, Diehm N, Regli C, Staub D. Rivaroxaban versus fondaparinux for thromboprophylaxis after endovenous laser ablation. J Vasc Surg Venous Lymphat Disord. 2017;5(6):817-823.