MPFF and the protection of venous valves
venous valves
Abstract
Increased venous pressure underlies all the clinical manifestations of chronic venous disorders. Venous hypertension is the result of incompetent venous valves in the superficial veins for most patients. A strong link between venous hypertension, valve failure and venous inflammation has been evoked through pharmacological studies and confirmed in a variety of animal models. A cascade of inflammatory reactions results in adverse changes in the venous valve and venous wall that eventually produce venous hypertension. Symptoms, telangiectasias, varicose veins, and eventually venous leg ulcers appear to be a consequence of the changes induced by venous hypertension. Treatment to inhibit inflammation and hamper venous hypertension may offer the greatest opportunity to prevent progression of the disease and related complications. Inflammation-dependent valve failure is considered as a target for drugs. MPFF, a venoactive drug containing purified micronized diosmin, hesperidin, linarin, isorhoifolin, and diosmetin at optimized doses, is the only drug with evidence on the preservation of valve structures in animal models and on the suppression of commissural transitory reflux that occurs in symptomatic patients after prolonged standing. MPFF also has been shown to protect the microcirculation in animal models. This is translated into clinical benefits, such as edema reduction, hematoma resorption after surgery, acceleration of ulcer healing, and amelioration of lymphatic drainage. The role of MPFF in the attenuation of the various elements of venous inflammation is now better known and would deserve a deeper exploration in the future.
Introduction
Venous valves were first described by Dutch physician Jacques Dubois, but their true function was later discovered by William Harvey.1
Most veins of the superficial and deep system in the lower extremities are equipped with a series of one-way bicuspid valves that open to allow blood to flow toward the heart and close to prevent reverse blood flow toward the feet. Particularly in the erect position, the venous valves are essential in assuring that blood flows in the correct direction, traveling against gravity and other pressures.2 Venous pathology develops when venous pressure increases and blood return is impaired by incompetent valves in the axial deep or superficial veins, perforator veins, or venous tributaries for most patients. Chronic venous disorders may also result from venous obstruction or a combination of both valve incompetence and obstruction. These mechanisms serve to produce global or regional venous hypertension, particularly with standing or walking.3 The subsequent macrocirculatory hemodynamic disturbances contribute to the large variety of clinical presentations seen in chronic venous disorders. Prolonged periods of venous hypertension in the legs, in turn, alter the microcirculation, resulting in dermal changes with hyperpigmentation, lipodermatosclerosis, and eventual ulceration.
The presentation of chronic venous disorders includes symptoms and signs. A recent large-scale epidemiological study has shown that the most commonly expressed chronic venous disorders–related symptoms include (in order of frequency): heaviness; leg pain; swelling sensation; nighttime cramps; sensation of “pins and needles” in the legs; and sensation of burning and itching.4 Signs of chronic venous disorders are described in the Clinical, Etiological, Anatomical, Pathophysiological (CEAP) classification and comprise telangiectasia, varicose veins, edema, skin changes, and healed or active venous leg ulcers.5
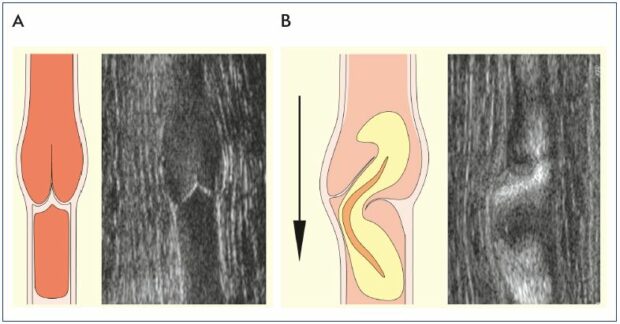
Figure 1. Visualization of competent and incompetent venous valves.
Competent (Panel A) and incompetent (Panel B) venous valves showing schematic and B-flow ultrasound images. In Panel B, the
valve sinus is distorted. The cusp above the dilatation is frozen and the adjacent cusp is prolapsed. The high-velocity retrograde
streaming deviates laterally above a prolapsing cusp.
From reference 6: Lane et al. Phlebolymphology. 2007;14:105-115. Image courtesy of the author.
A strong link is evoked between venous hypertension and valve failure
In most cases, venous hypertension is caused by reflux through incompetent venous valves (Figure 1).3,6
Examination of surgical specimens removed from limbs with chronic venous insufficiency, and more recently, the direct observation offered by angioscopy, has revealed lesions involving the venous wall, valvular annulus, and valve cusps.7,8 Failure of the valve and the valvular annulus is responsible for progression of the disease via maintenance and further increases in venous hypertension.
Immunohistochemical studies using a monoclonal antibody specific for monocytes and macrophages have demonstrated a monocyte/macrophage infiltration into the valve leaflets and venous wall of C2 patients with varicose veins.9 Monoclonal antibody studies have found leukocyte infiltration to be greater at both the base of the valve leaflets and in the proximal venous wall.
Venous valves have been found to be prominent in regions of low shear stress with venous eddies and recirculation (Figure 2).10,11 It may be that these phenomenon explain how the leukocytes are preferentially deposited in these regions. Ultimately, macrophages become the instrument of tissue damage that softens the venous wall and favors valve destruction.12 Venous valve failure, and the subsequent reflux that results in distal venous hypertension, may contribute to the sustained and chronic hypertension that is responsible for leukocyte activation at the endothelium and leukocyte destruction of skin and subcutaneous tissues at the ankle. In addition to leukocyte activation, increased mast cell infiltration into the venous wall may have a role in the development of varicose veins. Increased expression of intercellular adhesion molecule 1 (ICAM-1) and CD68 on the endothelial surface of venous walls in patients with venous insufficiency has been demonstrated and this increased expression may be related to the development of varicose veins.12 This finding suggests a continuing inflammatory reaction that is related to venous wall remodeling.13,14 Additionally, endothelial cells must be activated to allow leukocytes to migrate through the endothelial cell layer into the tissue.12 It is believed that endothelial stretching of the vein due to changes in blood flow and fluid shear stress may induce activation of the endothelium. Fluid shear stress is a key regulatory component of endothelial cells and a reduction in the rate of shear stress leads to enhanced adhesion of leukocytes to the endothelium.9
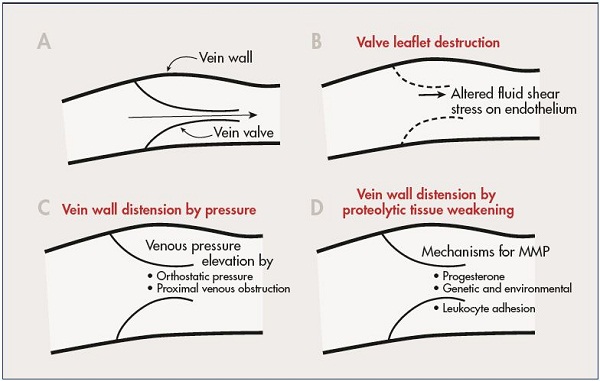
Figure 2. A schematic diagram illustrating selected mechanisms that may control inflammation of the vein wall and valve leaflet.
A normal vein valve and wall is shown in Panel A. Valve leaflets may be subject to inflammatory damage by alteration in
magnitude and direction of fluid shear stress on the endothelium (Panal B). Venous valves may become unable to close their
leaflets due to vein wall distension by elevated venous pressure (Panel C), or by weakening of the vein wall due to proteolytic
degradation of its extracellular matrix (Panel D).
Abbreviation: MMP, matrix metalloproteinases
From reference 11: Schmid-Schönbein. Medicographia. 2008;30:121-126. Image courtesy of the author.
Clinical observations are confirmed in animal models
Since the mechanisms responsible for venous valve failure in primary chronic venous disorders cannot be evidenced in vivo in human beings, animal models were set up for the experimental research. Lalka et al described a simple, reproducible model of hind-limb valve disruption in the greyhound.15 After this acute valve degeneration, animals developed an immediate increase in poststimulation segmental venous pressure that persisted for as long as 14 weeks. Despite demonstrating reflux in the segments with the disrupted valves, there was no extension into the tributaries and no evidence of varicose vein development. It was hypothesized that this was due to the relatively short hydrostatic column present in the quadruped hind limb.16
To elucidate the possible mechanisms for the valve remodeling in chronic venous disorders, another model involved developing an arteriovenous fistulae (AVF). Unfortunately, an arterialized pressure profile occurred in the distal veins, making this model unsuitable for studying this chronic disease. The combination of outflow obstruction and AVF to produce a model of sustained venous hypertension was developed by van Bemmelen17 and applied to the study of reflux development by Bergan’s team.18 In a series by Takase et al, rat saphenous vein valves were examined, in which femoral venous hypertension was elevated for a period of 3 weeks using the van Bemmelen model. In this model, venous reflux developed in response to venous hypertension around 100 mm Hg.
Examination of vein morphology revealed that valve failure occurred as a result of venous wall dilation and valve leaflet shortening to the point of incomplete valve closure and subsequent reflux. Assessment of the valves for molecular inflammatory markers revealed an enhanced leukocyte infiltration with granulocytes, monocytes, and T lymphocytes. In addition, the expression of P-selectin and ICAM-1, two endothelial cell membrane adhesion molecules, on the endothelial cells of the saphenous vein wall was increased.18 In this study, the leaflets were still able to close properly in the early stages after placement of the arteriovenous fistula, suggesting that pressure per se may not necessarily be the variable responsible for compromising the leaflets. However, at the time that the leaflets fail and reflux occurs, there was an observed reduction in the leaflet dimensions. A possible explanation of the sequence of events leading to morphological abnormalities in venous valves is that as the venous wall dilates, there may be a point reached when reflux develops across the leaflets. An abnormal fluid shear stress produced at the surface of the leaflets during venous reflux would be highly inflammatory for the endothelial cells on the valve leaflets and may trigger destruction of the leaflets, increasing venous hypertension and promoting a vicious circle of venous hypertension/ venous inflammation.
A new animal model of low flow and high pressure in veins to avoid the pitfalls of previous models is being developed by Bouskela’s team. The objectives of such a model are to achieve long periods of observation, study alterations in venous pressure over time, assess changes in microcirculatory parameters, and determine the inflammatory profile of the model. It will allow for an assessment of venous pressure and its evolution with time, and an exploration of the microcirculatory parameters with a Cytoscan® device and intravital microscopy.
Inflammation-dependent valve failure as a new drug target: the example of MPFF
Intervention in the inflammatory reaction that occurs as part of the progress of chronic venous disorders may be a new pharmacological target. For this reason, the models by Bergan and Bouskela have been used to assess the effect of MPFF*, a venoactive drug (VAD).
Chemical family of MPFF
MPFF is produced from a plant extract from the epicarp of Citrus aurantium var amara. It belongs to the chemical family of flavonoids that are included in the six main categories of venoactive drugs (Table I). MPFF contains purified micronized diosmin, hesperidin, linarin, isorhoifolin, and diosmetin at optimized doses.19 Each of the active ingredients in MPFF contribute to its action and explains its superior beneficial effect over other VADs on the reduction in capillary permeability.19
MPFF’s mode of action
The pharmacodynamic effects of MPFF and their clinical consequences are summarized in Table II.20-23
Preservation of venous valve structures
MPFF is the only VAD with evidence on the preservation of valve structures in animal models and on the suppression of commissural transitory reflux that occurs in symptomatic patients after prolonged standing.24 In two trials of pharmacological postoperative recovery for patients with varicose veins who underwent phlebectomy, MPFF helped attenuate postoperative pain and improve the quality of life.25-28
Protection of the microcirculation
Experimental in vivo models have been used to study the effect of drugs on the microcirculation. Microcirculatory preparations include hamster cheek pouch, hamster or mouse skinfold, rat or hamster mesentery, rat, hamster or mouse cremaster, etc.29 Numerous pharmacological trials have shown that VADs increase capillary resistance and reduce capillary filtration, resulting in the prevention of capillary leakage. MPFF has shown evidence for improved microvascular reactivity and functional capillary density (number of capillaries with flowing red blood cells per unit of tissue) after ischemia-reperfusion injury,30 and MPFF also induced a significant dose-related reduction in the increased permeability.31 Such protective microcirculatory properties of MPFF result in clinical benefits. In a recent meta-analysis, ten publications dated between 1975 and 2009 analyzed 1010 patients for the benefits of MPFF, hydroxyethylrutoside, ruscus extracts, and diosmin on edema reduction. Mean reduction in ankle circumference was -0.80±0.53 cm with MPFF, -0.58±0.47 cm with Ruscus extract, -0.58±0.31 cm with hydroxyethylrutoside, -0.20±0.5 cm with single diosmin, and -0.11±0.42 cm with placebo. The comparison between MPFF and other VADs on ankle reduction in edema was in favor of MPFF (P<0.0001).32
MPFF, used postsurgery after varicose vein stripping, helped decrease postoperative hematomas and helped accelerate their resorption.25-28
The complications of chronic venous disorders are related to chronic venous hypertension and are visualized in the skin, which is the final target of chronic venous hypertension. The hypertension is a cause of chronic inflammation manifested by persistent and sustained injury. Ultimately, the dermal capillary circulation is the most severely impaired in limbs with chronic venous insufficiency.
In a meta-analysis of 5 randomized controlled trials containing 723 C6 patients, MPFF demonstrates efficacy in healing venous ulcers when used as an adjunct treatment to compression therapy and appropriate local therapy, particularly for large (>5 cm2 in area) and/or persistent (>6-month duration) ulcers.33
Amelioration of lymphatic drainage
The draining function of lymphatic vessels is very important. Lymphatic vessels transport 4 L of efferent lymph into the bloodstream daily. The fluid turnover (including the volume of fluid reabsorbed in the lymph nodes) reaches up to twothirds of the total volume of interstitial fluid every 24 hours.34 The skin of the lower extremities contains a more dense and extensive network of lymphatic capillaries than the skin of the upper extremities.35 Due to orthostatism, lower extremities have higher filtration pressure and fluid influx. It is thought that the capacity for lymph transport in the lower extremities is greater in order to compensate for the higher influx of interstitial fluid caused by the effects of orthostatism and gravity. Spontaneous contractility of lymphatic vessels is utilized in lymph transport. Regular contractions of lymph vessels, at a frequency of 2 to 4 per minute, were observed in vitro. Spontaneous contractions of prenodal lymphatic vessels have been observed in human legs and were shown to drive the lymph flow.36 Internal extensions of lymphatic endothelial cells act as valves and guarantee a unidirectional lymph flow.34 Lymphatic dysfunction and structural damages to the lymphatic network are associated with varicose veins, and the subsequent lymph stasis and reduced lymph transportation lead to inflammation.37 This is associated with lipid accumulation in the media of the diseased veins, which may further damage adventitial lymphatic vessels.37
Pharmacological trials found that treatment with MPFF may help treat lymphedema by reducing protein and extracellular fluid accumulation,38 stimulating lymph contractility and flow,39 and reducing the excess protein in tissues with high protein edema. In a study investigating MPFF or placebo (n=48) over 6 months,40 the treatment group experienced a 7% volume reduction, while the placebo group experienced a 10% volume increase. Both groups experienced significant reduction in reported discomfort, but the treatment group also had a significant reduction in heaviness. In addition, MPFF was found to be efficacious in reducing edema volume in bancroftian filarial lymphoedema.41
Potent anti-inflammatory effect
Disturbed venous flow patterns and chronic venous inflammation are two interlinked phenomena. It is thought that mediators resulting from disturbed blood flow, and subsequent inflammation, have an important role in the occurrence of venous pain. Locally released proinflammatory mediators, resulting from hemodynamic changes and hypoxia, can activate nociceptors located in close contact with the microcirculation including the venous wall, the space between endothelial and smooth muscle cells of the media,42 and the perivenous space.
The primary activation site of venous and/or perivenous nociceptors may not happen in large venous vessels, which is suggested by the fact that pain is not closely correlated with objective parameters of varicose vein remodeling, incompetent venous valves, and inflammation. The efficacy of MPFF in the treatment of patients with symptoms of chronic venous disease has been widely evaluated in comparative and noncomparative clinical trials.20,23 There is substantial evidence from meta-analyses43 and the RELIEF study (Reflux assEssment and quaLity of lIfe improvEment with micronized Flavonoids), a large observational study,44 that MPFF is efficacious in relieving venous symptoms and lower limb edema. In the latest recommendations for the management of active venous ulcers, MPFF was assigned a grade 1B for adjuvant therapy, keeping in mind that MPFF is also capable of reducing associated pain.23
MPFF’s protective effect against inflammationrelated valve damage in chronic venous disease
In pharmacological studies
The ability of MPFF to mitigate or block the effects of chronic inflammation in the micro- and macrocirculation has been demonstrated in animal models. In a model of venous occlusion and reperfusion, the subsequent elevation of venous blood pressure increased the inflammatory cascade and tissue injury.45 In MPFF-treated animals, markers of inflammation were decreased in a dose dependent manner. MPFF also served to significantly reduce parenchymal cell death and leukocyte rolling, adhesion to postcapillary venules, and migration.46 Important data supporting the protective effect of MPFF on the macrocirculation have been provided by Takase et al.47 In animals treated with MPFF, there was a significant, dose-dependent reduction in the reflux rate (Figure 3). MPFF also reduced several indicators of the inflammatory reaction in a dose-dependent manner, including leukocyte infiltration, expression of P-selectin and ICAM-1, and the level of apoptosis. By delaying or blocking the inflammatory reaction, these data suggest that MPFF may delay the development of reflux and suppress damage to valve structures in the rat model of venous hypertension. These observations were recently confirmed in a new study using the same animal model. The administration of MPFF reduced edema and fistula blood flow produced by the acute AVF. MPFF also reduced granulocyte and macrophage infiltration into the valves, which is consistent with the previous study.48
In clinical trials
A 2-month treatment with MPFF at 1000 mg/day resulted in the elimination of transitory commissural reflux observed in patients presenting with subjective leg symptoms without visible signs of chronic venous disorders, the so-called C0s patients (Figure 4).24 Transitory reflux elimination was paralleled with pain relief and quality of life amelioration. In this trial, consecutive C0s patients were enrolled and assessed for the following: (i) symptom intensity using the visual analog scale (VAS); (ii) quality of life with the Chronic Venous Insufficiency quality of life Questionnaire (CIVIQ-20); and (iii) saphenous reflux duration and saphenous vein diameter by a Duplex scan examination performed twice a day (morning and evening). A total of 41 C0s patients were enrolled in the study; and of these patients, 15 had no reflux in either the morning or evening and 26 had transitory evening reflux with 22 being commissural and 4 intervalvular. The saphenous vein diameter was greater in the subgroup of patients with transitory reflux compared with patients without reflux (P<0.05). After MPFF treatment, there was a trend toward a reduction in intervalvular reflux length (despite being nonsignificant), while transitory commissural refluxes (n=22) no longer appeared. Additionally, vein diameter returned to normal values. These results mirror the protective effect of MPFF on venous valve structures.
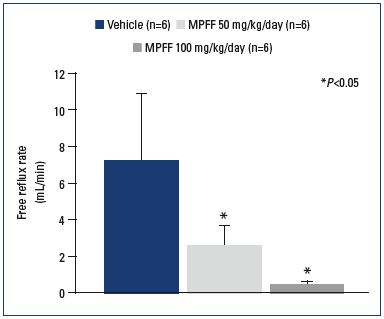
Figure 3. Reflux flow rates across the valve of the saphenous
vein.
Reflux flow rates across the saphenous venous valve measured
after 3 weeks of venous hypertension in control (vehicle)- and
MPFF-treatment groups at a dose of 50 and 100 mg/
kg/day. N is the number of rats in each treatment group. P<0.05
compared with control.
From reference 47: Takase et al. Eur J Vasc Endovasc Surg. 2004;28:484-
493. © 2004, Elsevier Ltd.
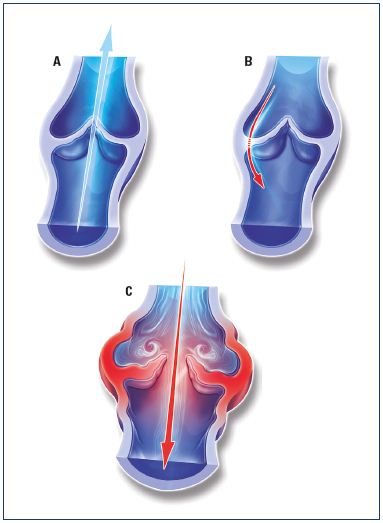
Figure 4. Illustrations of venous valves with and without reflux.
Illustrations of a normal venous valve without reflux (Panel A), a
valve with a nonpathological commissural reflux usually seen in
the evening after being in a prolonged upright position (Panel
B), and a valve with a pathological intervalvular reflux (Panel C).
Modified from reference 24: Tsoukanov et al. Phlebolymphology.
2015;22:18-24. Image courtesy of the author.
Venous valve protection opens perspectives for targeted pharmacological interventions
The practical purpose of elucidating the molecular steps involved in the development of valve lesions is to intervene with a targeted treatment. Studies have focused on available molecules known to modify the sequence of events involving leukocyte adhesion, endothelial interaction, activation, and migration, and the subsequent associated valvular damage in large veins, mainly the great saphenous vein. However, studies on the pathophysiology of chronic venous disorders have not yet acknowledged that this sequence of events is not limited to large veins including the saphenous veins, but extends down to venules, where valves and microvalves play important roles in venous hemodynamics. We know from recent findings that the majority of microvalves in lower limbs are present within channels less than 100 μm in luminal diameter.49 The role that microvalves play is still unclear and their location and arrangement in normal lower limbs suggest that they prevent blood flow into the capillary bed (Figure 5). This has been evidenced by Philips, who found no difference between lower limbs with venous ulcers and normal limbs with respect to the number and density of microvalves. However, microvalves in diseased limbs were stretched and incompetent, allowing retrograde flow from large veins into the dermal capillary bed.49 Vincent and coworkers proposed two hypotheses: (i) degenerative changes in very small veins in leg skin may be related to the appearance of telangiectasias, reticular veins, and corona phlebectatica; and (ii) valve incompetence in both larger proximal vessels and small superficial veins, at the level of microvalves, would account for the appearance of severe skin changes in the event of venous insufficiency.50
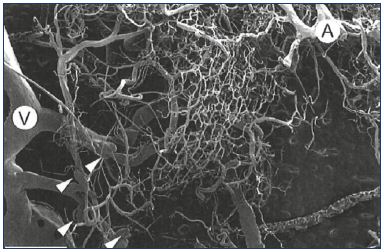
Figure 5. Arterial and venous capillary bed network.
Capillary bed network associated with an artery (A) and a vein
(V). Four “microvalves” are visible (arrowheads). Scale bar=2
mm.
From reference 49: Phillips et al. Clin Anat. 2004;17:55-60. © 2003, Wiley-
Liss, Inc.
MPFF currently possesses the most appropriate profile to protect venous valves and perhaps microvalves, even if its role remains to be more deeply explored in vivo.
*Also registered as Ardium®, Alvenor®, Arvenum® 500, Capiven®, Detralex®, Elatec®, Flebotropin®, Variton®, Venitol®
1. Caggiati A. The venous valves of the lower limbs. Phlebolymphology. 2013;20:87-95.
2. Eberhardt RT, Raffetto JD. Chronic venous insufficiency. Circulation. 2014;130:333- 346.
3. Bergan JJ, Schmid-Schönbein GW, Coleridge-Smith PD, Nicolaides AN, Boisseau MR, Eklof B. Chronic venous disease. N Engl J Med. 2006;355:488- 498.
4. Rabe E, Guex JJ, Puskas A, Scuderi A, Fernandez Quesada F; VCP Coordinators. Epidemiology of chronic venous disorders in geographically diverse populations: results from the Vein Consult Program. Int Angiol. 2012;31:105-115.
5. Rabe E, Pannier F. Clinical, aetiological, anatomical and pathological classification (CEAP): gold standard and limits. Phlebology. 2012;27(suppl 1):114- 118.
6. Lane RJ, Graiche JA, Cuzzilla ML, Coroneos JC. Incompetent venous valves: ultrasound imaging and exo-stent repair. Phlebolymphology. 2007;14:105-111.
7. Corcos L, De Anna D, Dini M, Macchi C, Ferrari PA, Dini S. Proximal long saphenous vein valves in primary venous insufficiency. J Mal Vasc. 2000;25:27-36.
8. Van Cleef JF, Hugentobler JP, Desvaux P, Griton P, Cloarec M. Endoscopic study of reflux of the saphenous valve. J Mal Vasc. 1992;17:113-116.
9. Ono T, Bergan JJ, Schmid-Schönbein GW, Takase S. Monocyte infiltration into venous valves. J Vasc Surg. 1998;27:158- 166.
10. Lurie F, Kistner RL, Eklof B, Kessler D. Mechanism of venous valve closure and role of the valve in circulation: a new concept. J Vasc Surg. 2003;38:955-961.
11. Schmid-Schönbein GW. Triggering mechanisms of venous valve incompetence. Medicographia. 2008;30:121-126.
12. Takase S, Bergan JJ, Schmid-Schönbein GW. Expression of adhesion molecules and cytokines on saphenous veins in chronic venous insufficiency. Ann Vasc Surg. 2000;14:427-435.
13. Jacobs MP, Badier-Commander C, Fontaine V, Benazzoug Y, Feldman L, Michel JB. Extracellular matrix remodeling in the vascular wall. Pathol Biol (Paris). 2001;49:326-332.
14. Badier-Commander C, Jacobs MP, Michel JB. Varicose remodeling (in French). Med Ther. 2000;6:718-723.
15. Lalka SG, Unthank JL, Dalsing MC, Cikrit DF, Sawchuk AP. Venous hemodynamics in a chronic venous valvular insufficiency model. Arch Surg. 1990;125:1579-1583.
16. Jones GT. Animal models in chronic venous disease. Medicographia. 2008;30:154-156.
17. van Bemmelen S, Hoynck van Papendrecht AA, Hodde K, Klopper PJ. A study of valve incompetence that developed in an experimental model of venous hypertension. Arch Surg. 1998;121:1048-1052.
18. Takase S, Pascarella L, Bergan J, Schmid- Schönbein GW. Hypertension-induced venous valve remodeling. J Vasc Surg. 2004;39:1329-1334.
19. Paysant J, Sansilvestri-Morel P, Bouskela E, Verbeuren TJ. Different flavonoids present in the micronized purified flavonoid fraction (MPFF at a dose of 500 mg) contribute to its anti-hyperpermeability effect in the hamster cheek pouch microcirculation. Int Angiol. 2008;27:81-85.
20. Lyseng-Williamson KA, Perry CM. Micronised purified flavonoid fraction: a review of its use in chronic venous insufficiency, venous ulcers and haemorrhoids. Drugs. 2003;63:71-100.
21. Perrin M, Ramelet AA. Efficacy of venoactive drugs in primary chronic venous disease. Survey of evidence, synthesis and recommendations. In: Bergan JJ, Bunke N, eds. The Vein Book. 2nd ed. New York, NY: Oxford University Press; 2014:514-527.
22. Perrin M, Ramelet AA. Pharmacological treatment of primary chronic venous disease: rationale, results and unanswered questions. Eur J Vasc Endovasc Surg. 2011;41:117-125.
23. Nicolaides A, Kakkos S, Eklof B, et al. Management of chronic venous disorders of the lower limbs – guidelines according to scientific evidence. Int Angiol. 2014;33:87-208.
24. Tsoukanov YT, Tsoukanov AY, Nikolaychuk A. Great saphenous vein transitory reflux in patients with symptoms related to chronic venous disorders, but without visible signs (C0s), and its correction with MPFF treatment. Phlebolymphology. 2015;22:18-24.
25. Pokrovsky AV, Saveljev VS, Kirienko AI, et al. Surgical correction of varicose vein disease under micronized diosmin protection (results of the Russian multicenter controlled trial DEFANS). Angiol Sosud Khir. 2007;13:47-55.
26. Pokrovsky AV, Saveljev VS, Kirienko AI, et al. Stripping of the great saphenous vein under micronized purified flavonoid fraction (MPFF) protection (results of the Russian multicenter controlled trial DEFANCE). Phlebolymphology. 2008;15:45-51.
27. Veverkova L, Kalac J, Jedlicka V, et al. Analysis of surgical procedures on the vena saphena magna in the Czech Republic and an effect of Detralex during its stripping [article in Czech]. Rozhl Chir. 2005;84:410-412.
28. Veverkova L, Kalac J, Jedlicka V, et al. Analysis of the various procedures used in great saphenous vein surgery in the Czech Republic and benefit of MPFF at a dose of 500 mg to postoperative symptoms. Phlebolymphology. 2006;13:195-201.
29. Virgini-Magalhaes CE, Bottino DA, Bouskela E. Microcirculation and chronic venous insufficiency: from production of pharmacological models to discovery of new therapies. Phlebolymphology. 2001;35:16-19.
30. Bouskela E, Cyrino FZ, Lerond L. Effects of oral administration of different doses of purified micronized flavonoid fraction on microvascular reactivity after ischemia/ reperfusion in the hamster cheek pouch. Br J Pharmacol. 1997;122:1611-1616.
31. Cyrino FZ, Bottino DA, Lerond L, Bouskela E. Micronization enhances the protective effect of purified flavonoid fraction against postischaemic microvascular injury in the hamster cheek pouch. Clin Exp Pharmacol Physiol. 2004;31:159- 162.
32. Allaert FA. Meta-analysis of the impact of the principal venoactive drugs agents on malleolar venous edema. Int Angiol. 2012;31:310-315.
33. Coleridge-Smith P, Lok C, Ramelet AA. Venous leg ulcer: a meta-analysis of adjunctive therapy with micronized purified flavonoid fraction. Eur J Vasc Endovasc Surg. 2005;30:198-208.
34. Rovenská E, Rovenský J. Lymphatic vessels: structure and function. Isr Med Assoc J. 2011;13:762-768.
35. Stanton AW, Patel HS, Levick JR, Mortimer PS. Increased dermal lymphatic density in human leg compared with forearm. Microvasc Res. 1999;57:320-328.
36. Olszewski WL, Engeset A. Intrinsic contractility of prenodal lymph vessels and lymph flow in human leg. Am J Physiol. 1980;239:H775-H783.
37. Tanaka H, Zaima N, Sasaki T, et al. Loss of lymphatic vessels and regional lipid accumulation is associated with great saphenous vein incompetence. J Vasc Surg. 2012;55:1440-1448.
38. Casley-Smith JR, Casley-Smith JR. The pathophysiology of lymphedema and the action of benzo-pyrones in reducing it. Lymphology. 1988;21:190-194.
39. Clement DL. Management of venous edema: insights from an international task force. Angiology. 2000;51:13-17.
40. Pecking AP, Fevrier B, Wargon C, Pillion G. Efficacy of MPFF at a dose of 500 mg in the treatment of lymphedema (secondary to conventional therapy of breast cancer). Angiology. 1997;48:93-98.
41. D as L, Subramanyam Reddy G, Pani S. Some observations on the effect of micronized purified flavonoid fraction (MPFF) in bancroftian filarial lymphoedema. Filaria J. 2003;2:5.
42. Vital A, Carles D, Serise JM, Boisseau MR. Evidence for unmyelinated C fibers and inflammatory cells in human varicose saphenous veins. Int J Angiol. 2010;19:e73-e77.
43. Martinez MJ, Bonfill X, Moreno RM, Vargas E, Capella D. Phlebotonics for venous insufficiency. Cochrane Database Syst Rev. 2005;(3):CD003229.
44. Jantet G. Chronic venous insufficiency: worldwide results of the RELIEF study. Reflux assEssment and quaLity of lIfe improvEment with micronized Flavonoids. Angiology. 2002;53:245-256.
45. Takase S, Lerond L, Bergan JJ, Schmid- Schönbein GW. Enhancement of reperfusion injury by elevation of microvascular pressures. Am J Physiol Heart Circ. 2002;282:H1387-H1394.
46. Takase S, Delano FA, Lerond L, et al. Inflammation in chronic venous insufficiency: is the problem insurmountable? J Vasc Res. 1999;36(suppl 1):3-10.
47. Takase S, Pascarella L, Lerond L, Bergan JJ, Schmid-Schönbein GW. Venous hypertension, inflammation and valve remodeling. Eur J Vasc Endovasc Surg. 2004;28:484-493.
48. Pascarella L, Lulic D, Penn AH, et al. Mechanisms in Experimental Venous Valve Failure and their Modification by MPFF at a dose of 500 mg. Eur J Vasc Endovasc Surg. 2008;35;102-110.
49. Phillips MN, Jones GT, van Rij AM, Zhang M. Micro-venous valves in the superficial veins of the human lower limb. Clin Anat. 2004;17:55-60.
50. Vincent JR, Jones GT, Hill GB, van Rij AM. Failure of microvenous valves in small superficial veins is a key to the skin changes of venous insufficiency. J Vasc Surg. 2011;54:62S-69S.