New diagnostic modalities in lymphedema
Abstract
Introduction: Lymphedema is a chronic, progressive, and debilitating disease. An early and accurate diagnosis and treatment is very important to alter the normal progression of the disease. When lymphedema is diagnosed late, the options for treatment are diminished as fibrous tissue is formed. Until recently, lymphoscintigraphy was considered the gold-standard diagnostic technique; however, other new and renewed diagnostic tools have emerged. This article provides an overview of the available diagnostic tools based on the findings from a literature search.
Methods: A literature search for each of the diagnostic tools was performed.
Results: Diagnostic tools can be divided into noninvasive and invasive techniques. Ultrasonography is a noninvasive technique that can identify lymphedema by looking at the thickness of the skin and echogenicity. Magnetic resonance and computed tomography scans show similar alterations of the skin, but can detect other causes for lymphedema. Near-infrared fluorescence imaging gives a realtime image of the superficial lymphatic transport, evaluates the severity of the lymphedema, and determines the functionality of the lymphatics.
Conclusions: This literature search showed that there are many techniques available besides lymphoscintigraphy. A good clinical examination and history is a critical first step. Ultrasonography is a noninvasive and inexpensive technique that can be used to diagnose lymphedema based on tissue changes. Lymphoscintigraphy and near-infrared fluorescence imaging can diagnose lymphedema and visualize the lymphatic architecture. Near-infrared fluorescence imaging can guide treatment and evaluate the response to treatment.
Introduction
Lymphedema is a chronic, progressive, and debilitating disease. It decreases patients’ quality of life due to an enlargement of the diseased limb, a decrease in mobility, and recurrent infections.1,2 Edema is defined as the presence of an excess of interstitial fluid, which happens when the microvascular filtration rate is higher than the drainage. In the past, it was thought that venous reabsorption played the most important role in reducing interstitial fluid (the Starling principle)3; however, recent research has shown that there is no reabsorption by the venous end of the capillaries. Instead, there is filtration along the entire capillary bed and the fluid regulation depends on the lymphatic transport.4 Fluid drains from the capillaries into the precollectors and the collectors. The collectors are lined with smooth muscle cells, and have lymphangions or vessel segments that are separated by valves, and are responsible for the unidirectional flow as they gradually contract and push the lymph forward.
Lymphedema can be divided into primary and secondary lymphedema. In primary lymphedema, there is an anomaly in the development of the lymphatic system, giving rise to structural and/or functional abnormalities in the lymphatic drainage. Until recently, a classification was used according to age of onset: congenital (from birth or shortly after), praecox (occurring before the age of 35), and tarda (occurring after the age of 35). Lymphangiographic findings, such as aplasia, hypoplasia, and hyperplasia, have been added to the age classification.5 A better understanding of the genes involved in the development of lymphedema has shown that certain genetic forms of primary lymphedema can present later in life. As more causal genes are identified, a different classification arises according to the patient’s phenotype. Primary lymphedema can also be part of a broader genetic syndrome, such as Turner syndrome or Noonan syndrome, or associated with overgrowth anomalies, such as Proteus syndrome.3
Most lymphedema cases are caused by secondary lymphedema,6 which results from damage or malfunction of the lymphatic transport system, such as an obstruction of the lymphatic transport (previous surgery, infection, malignancies) or an overload of interstitial fluid (eg, advanced venous insufficiency). For example, the incidence of upper extremity lymphedema after breast cancer treatment (with axillary lymph node dissection) ranges from 9% to 41%,7 and, when an inguinofemoral lymph node dissection is performed, approximately 20% of patients with melanoma, gynecological cancer, or prostate cancer will develop lymphedema.7
In most cases, the diagnosis of lymphedema can be made by obtaining the patient’s history and conducting a good clinical examination, which consists of age of onset, medication, travel to tropical countries, all causes of secondary lymphedema, family history, etc. As part of the diagnostic workup, systemic causes of edema need to be excluded (eg, heart failure, nephrotic syndrome, pulmonary hypertension, hypothyroidism, deep vein thrombosis, and chronic venous insufficiency). Lymphoscintigraphy has replaced lymphangiography as the standard for the diagnosis of lymphedema; however, other promising techniques have recently been developed.
Clinical evaluation
Lymphedema can progress from a soft, pitting edema to a hard, fibrotic, nonpitting edema, which is because lymph stasis will cause extravasation of fluid in the interstitium and promote lipogenesis, fibrosis, inflammation, lymphangiogenesis, and immunosuppression.8 However, if diagnosis occurs early, the progression to a fibrotic edema can be altered.
According to history and clinical examination, a clinical stage system can be used, based on the International Society of Lymphology.1,9 Stage 0 refers to a subclinical state, where edema is not yet visible despite impaired lymphatic transport. Stage I refers to an early accumulation of fluid with a high protein content, whereby the edema subsides by elevating the limb. Stage IIa represents swelling that does not subside when the limb is elevated and pitting is manifest. In stage IIb, no pitting is visible and fibrosis together with fat emerges. Stage III, also known as “lymphostatic elephantiasis,” is the most advanced form with skin abnormalities and further fibrosis of the tissue. This staging system is not complete because it does not take into account why some patients develop a soft, fatty lymphedema and others develop a hard fibrotic one. These differences are also seen when circumferential suction assisted lipectomy is performed, where more fat is removed than fluid.10 This fluid and fatty tissue can be measured with several clinical tools and a clinical examination.
Measuring the skin fold (Stemmer sign) can provide an estimation of the thickness of the cutis, which is a typical sign of lymphedema. The Stemmer sign is more often visible at the foot with primary lymphedema than with secondary lymphedema where the edema is more often seen proximal. The existence of pitting plays an important role in determining the clinical stage, and it can be determined with the “pitting sign” (Figure 1). Puffiness of the forefoot can be noted. In more advanced cases, skin abnormalities, such as hyperkeratosis, papillomatosis, lymph vesicles, and eczema, can occur. The chronic accumulation of lymph can also promote infection.8
Volume can be assessed using different tools. Circumference tape measurements, with measurement intervals varyingfrom 3 to 12 cm, and different anatomical points can be used to calculate volume (Figure 2).11,12 Perometry, an optoelectric device, uses infrared light to image the external surface of the limb and calculate its volume. This measurement tool is not widely available and not easy to use accurately.13 Volumetric measurements (volumetry) can be done with water displacement, where the extremity is immerged in a container of water, the amount of the displaced volume represents the volume of the limb.14,15 Bioimpedancy spectroscopy (BIS), a noninvasive method to measure body composition, uses electric resistance to measure the amount of extracellular fluid.16
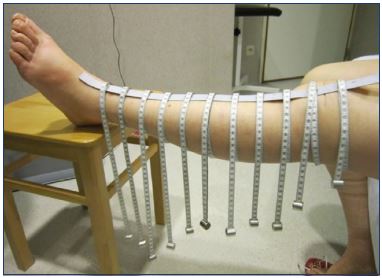
Figure 2. Tape measurements.
From reference 11: Devoogdt et al. Int Angiol. 2010;29(5):401-
407. © 2010, N. Devoogdt
A recent review analyzed different measurement instruments used in clinical studies. They concluded that BIS, volumetry, perometry, and tape measurements can be recommended in clinical practice.12 The usefulness of measurement tools depends on the stage of the edema, eg, BIS can detect edema earlier than volume measurements,17 BIS and perometry are more expensive than water volumetry and tape measurements, volumetry and perometry are difficult to use for measuring midline lymphedema (head, trunk, genitalia). The suitability of the tool also depends on the feasibility and costs.12
Lymphography
Lymphangiography or lymphography is the oldest visualization technique that was invented by Kinmonth et al.5 For this method, patent blue is injected intradermally to visualize the lymphatics, then a cannula is inserted through a microsurgical incision, and subsequently, an oil-soluble iodinated contrast medium is infused into the lymphatic vessel. Other methods involve an indirect uptake of an intradermally administered water-soluble iodinated contrast agent through the lymph capillaries and into the vessels.18 These techniques are not easy to perform, meaning that they rarely used. In addition, an infusion of a contrast agent (as described by Kinmonth) or a puncture directly into a lymph node is still used in combination with a computed tomography (CT) or magnetic resonance imaging (MRI) to visualize leakages from the central lymphatics, identify lymphatic malformations, or diagnose and treat chylous reflux.19 Therefore, lymphography has, in combination with CT or MRI, some diagnostic applications.
Ultrasonography
Ultrasonography can be used to visualize enlarged iliac, axillary, para-aortal, and paracaval lymph nodes and detect abdominal masses that are obstructing lymphatic flow. This technique is helpful to identify abnormal lymph nodes in the head and neck region. It is a simple, noninvasive technique; however, cannot visualize the lymphatic architecture. High frequency ultrasonography is a noninvasive tool that can be used to evaluate the skin and subcutaneous thickness and echogenicity. Reticular patterns with little or no reflex can be seen, which corresponds to edema-filled gaps between fat lobules or to fat cells themselves. Using this technique, the postoperative evolution of the thickness of the cutis and subcutis was assessed in a healthy arm as well as in an arm with lymphedema. Ultrasound can be used as an adjunctive diagnostic tool, but not as a separate tool.20 Suehiro et al showed that the thickness of the skin and subcutaneous tissue as well as the echogenicity correlated well with the International Society of Lymphology staging system.21
Ultrasonography can also be used to differentiate lipedema from lymphedema.22 The results of the study by Naouri et al22 confirmed that lipedema is due to an increase in hypodermal tissue with no true dermal edema. Lipedema showed dermal echogenicity that was similar to normal skin. In lymphedema, the thickness of the skin and dermal hypoechogenicity is increased, especially in the distal part of the extremities for primary lymphedema. Duplex ultrasonography can be used to evaluate the function of venous valves (primary venous reflux) and exclude deep vein thrombosis or postthrombotic syndrome as a cause of the edema. In some cases of primary lymphedema, such as Milroy disease, venous reflux coexists.23 In conclusion, ultrasonography is a good tool to diagnose lymphedema and assess the severity of the disease.24
CT and MRI scans
CT and MRI can be useful to visualize the lymph nodes, detect abnormalities, such as enlarged lymph nodes, visualize vascular malformations, or differentiate the causes of lymphatic obstruction in secondary lymphedema.23 As with ultrasonography, the affected skin and the subcutaneous tissue layers can be imaged. Contrast-enhanced MRI provides anatomical and functional information on the lymphatic drainage of the limb. Pathological findings on MRI include vessel dilatation, collateral formation, delayed vessel filling, delayed lymph node enhancement, honeycombing (characteristic distribution of edema within the epifascial compartment), and dermal backflow.25 MRI and CT are good tools to diagnose lymphedema and assess the severity of the disease. MRI lymphangiography has a good application when monitoring the treatment of chylous syndromes.19
Lymphoscintigraphy
The most commonly used diagnostic tool for examining extremity lymphedema is lymphoscintigraphy. This technique, first described by Sherman and Ter-Pogossian in 1953, directly images the lymphatic system.26 A radionuclide (99mTc-labeled tracer) is intradermally injected in the patient’s foot or hand and the uptake of the tracer into the lymphatic tissue is followed with sequential gamma imaging.27,28 This technique is recommended by the International Society of Lymphedema and the American Venous Forum guidelines.1 This technique not only provides dynamic imaging of the lymphatics and the lymph nodes, but also provides semiquantitative data on radionuclide transport. It also records the movement of the colloid from the injection site, the transition time to the knee, groins, or axilla, the absence or presence of major lymphatic collectors, the number and size of vessels and nodes, the presence of dermal backflow (reflux to the capillary network), the presence of collaterals, and symmetric activity with the opposite side.1
Signs of lymphedema include poorly visualized lymphatic collectors, delayed nodal enhancement, and dermal backflow. Lymphoscintigraphy can be used to diagnose lymphedema and estimate the severity of the lymphedema.28 In addition, it can be used to assess the efficacy of the treatments for lymphedema, eg, after lymphovenous anastomosis or lymph node transfer.29 Although lymphoscintigraphy is still the gold-standard technique, there is no standardization regarding the radiotracer, radioactivity doses, different injection volumes, intracutaneous vs subcutaneous injection, number of injections, different protocols of passive and active physical activity, varying imaging times, static and/or dynamic techniques.23,30
Near-infrared fluorescence imaging
Near-infrared fluorescence imaging is an alternative where a fluorescent agent is injected in place of the radionuclide; the main advantage is that this is a real-time image, as uptake is observed immediately. For humans, fluorescein sodium and indocyanine green (ICG) are used. Fluorescence is a phenomenon of light emission with a certain wavelength from a material when it is irradiated by light from another wavelength. The irradiated and emitted lights are called excitation light and fluorescence light, respectively.31 Fluorescein sodium is excited by visible light and ICG by near-infrared light. ICG has already been used for hepatic clearance and ophthalmological indications. The fluorescence technique is also used for sentinel node mapping in cancer surgeries.32,33 Fluorescein sodium is mainly used for microlymphangiography.24
After intradermally injecting ICG in the hand or foot, excitation light penetrates and fluorescence is observed with a photodynamic eye (PDE) camera to a maximum depth of 2 cm (Figure 3) with the frequency-domain photon migration technique (FDPM imager) to a maximum depth of 3 to 4 cm. Fluorescence from ICG is weak, but it becomes stronger when it is combined with plasma proteins and illuminated with an appropriate wavelength (Figure 4).34 The near-infrared light penetrates the human tissue well, but it is still absorbed to a certain extent by hemoglobin. Therefore, veins can be observed even without ICG.33
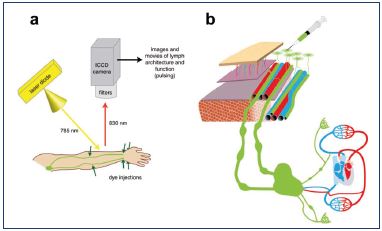
Figure 4. Fluorescence imaging.
A. The emitted excitation light. B. Indocyanine green (ICG) is
injected intradermally, captured by the capillary network, and
transported in precollector and collectors.
From reference 31: Aldrich et al. Biomed Opt Express.
2012;3(6):1256-1265. © 2012, Optical Society of America.
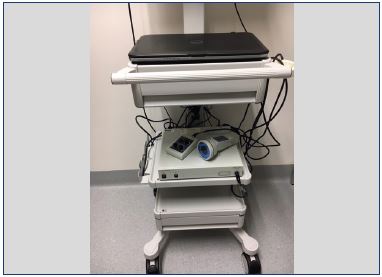
Figure 3. Photodynamic eye camera.
From reference 11: Chi et al. Theranostics. 2014;4(11):1072-
1084. © 2014, Ivyspring International Publisher.
Eva Sevick-Muraca’s group performed a series of studies using this technique and a summary of the human studies is provided in the following paragraphs.33 First, they optimized the technique in animals, moving to normal volunteers and finally to patients (upper and lower limb). Marshall et al35 gave an update on near-infrared fluorescence imaging in humans with a 0.1 mL injection of ICG at numerous sites and a FDPM imager to capture the energy.
Rasmussen et al36 described the use of two custom built fluorescence imaging systems to try to measure the velocity, defined as the distance over the travel time of the fluorescent packet. A propulsion event was defined as the time when the lymphangion empties. The contractions (pumping actions) of the lymphangions create intermittent suction of lymph from one lymphangion to another. The mean propulsion rate is the total number of fluorescent packets over the total time spent imaging (Figures 5 and 6).
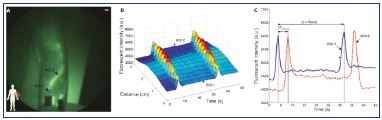
Figure 5. Quantification of the lymphatic flow.
Fluorescence intensity from one point to another.
From reference 36: Rasmussen et al. Transl Oncol. 2010;3(6):362-
372. © 2010, Neoplasia Press, Inc.
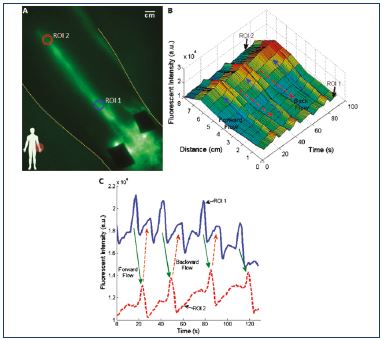
Figure 6. Lymphatic reflux.
Fluorescence intensity from one point to another and reflux.
From reference 36: Rasmussen et al. Transl Oncol. 2010;3(6):362-
372. © 2010, Neoplasia Press, Inc.
Tan et al37 performed a feasibility study to assess the lymphatic system after manual lymphatic drainage. The study used two custom-built fluorescence-imaging systems to measure velocity, propulsion events, and period between peaks. Ten patients with stage I and II lymphedema and twelve healthy volunteers were recruited. ICG was injected, and, after 30 minutes of imaging, manual lymphatic drainage was performed for 25 minutes, followed by a period of imaging during manual lymphatic drainage for 30 minutes. The results from the arm showed an increase in the velocity after manual lymphatic drainage (significant in the control and the asymptomatic group) and a decrease in the period between peaks (significant in the control group). The results from the legs showed an increase in the velocity after manual lymphatic drainage (significant in the control group) and a decrease in the period between peaks (nonsignificant in both the control group and the asymptomatic group). The stimulation of the lymphatic system was not as large in the symptomatic group as in the control group, probably because there are fewer lymph vessels to stimulate. With this technique, malfunction in the lymphangion contractions can result in slow uptake or passive filling of the ICG in the initial lymphangion, which can create an insufficient pressure gradient, reflux, or dermal backflow of the ICG.
Several schools in Japan have reported on their experience with near-infrared fluorescence. Unno et al38 first published a preliminary experiment with fluorescence lymphography in patients with secondary lymphedema (16 extremities); 0.2 mL ICG was injected and a PDE camera system was used to capture whole limb panoramic images. Four abnormal patterns were described: dermal backflow, extended dermal backflow in the foot, dilated lymph channels with proximal obliteration, and diffuse glittering (twinkling like the Milky Way). In one case, different patterns were observed in the same extremity. The authors concluded that ICG fluorescence is a good qualitative examination. This “Milky Way” reflects the stage where the lymphatic capillaries are filled with ICG and leakage in the interstitial space exists. Several dots with high concentration of ICG can be seen.
Suzuki et al39 investigated the effect of surgical treatment on lymph flow in the lower limbs of patients with varicose veins. A total of 39 patients were included and 15 healthy volunteers were used as controls, ICG lymphography was performed before and 6 months after great saphenous vein surgery. Transit time in a standing position was defined as the time until the ICG reached the knee (from dorsum foot to midpoint patella). They concluded that the transit time was longer in higher clinical, etiological, anatomical, and pathophysiological (CEAP) classes and the transit time was shorter after surgery.
Yamamoto et al40-44 performed several studies and described a severity staging of the dermal backflow image in secondary lymphedema of the upper and lower limb. In normal individuals, a linear pattern was observed. When dermal backflow exists, three stages are identified: splash, stardust, and diffuse (Figure 7). A splash pattern occurs when there is dermal backflow into the lymphatic capillaries, a stardust pattern represents the Milky Way, showing that some extravasation of ICG in the interstitial space has already occurred (Figure 8),45 and a diffuse pattern shows a fusion of all of the stars. In another study, a good correlation between the Campisi clinical classification and the dermal backflow patterns was observed.42
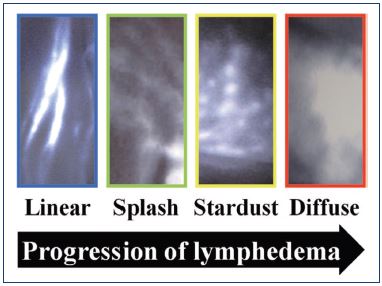
Figure 7. Classification of severity.
From reference 42: Yamamoto et al. Plast Reconstr Surg.
2011;128(4):941-947. © 2017, American Society of Plastic
Surgeons.
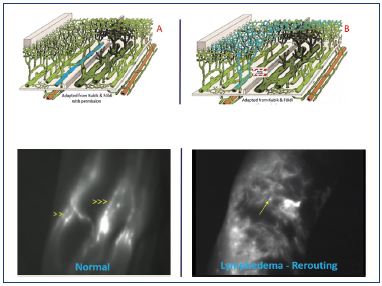
Figure 8. Superficial lymphatic network in the crook of the elbow.
A. Normal: linear pattern. B. Dermal backflow: stardust pattern.
From reference 45: Belgrado et al. Lymphat Res Biol.
2016;14(2):70-77. © 2016, Belgrado et al.
In a prospective trial that included 29 patients with lymphedema after gynecological cancer, Mihara et al46 compared the sensitivity and specificity of ICG lymphography with scintigraphy in secondary lower limb lymphedema. Another study, from a group in Chiba, Japan,47 compared the same diagnostic tools in patients with secondary lymphedema of the upper and lower limbs and idiopathic edema (suspected primary lymphedema). Both studies concluded that ICG lymphography is more accurate and that it is useful for assessing the indications for surgery (abnormal pattern progresses or extension into the next stage). Compared with lymphoscintigraphy, this technique does not use a radionuclide, has a lower cost, and shows a benefit for an early detection of lymphedema.47 However, standardization and further validation is needed before this technique can be used as a reproducible tool to characterize lymphedema patients. Furthermore, quantitative methods of the near-infrared fluorescence at all stages of lymphedema have not yet been developed. In this regard, it can be questioned whether it is possible to quantify the transport in a severe stage of lymphedema where there is not a linear structure to measure the velocity or propulsion rate.
Conclusion
Until recently, the gold-standard technique to confirm the presence of lymphedema has been lymphoscintigraphy; however, many newer diagnostic tools have become available. Clinical examination and history still play an important role, but noninvasive methods, such as ultrasonography are good additional diagnostic tools. A pitfall of ultrasonography is that it does not provide information on the functionality of the lymphatic transport or the architecture of the transport. Near-infrared fluorescence imaging seems a good tool to visualize the superficial lymphatic architecture, to assess the severity of lymphedema according to the different images, and to use it as a guide for manual lymphatic drainage. Compared with lymphoscintigraphy, this technique does not use a radionuclide, has a lower cost, and shows a benefit for an early detection of lymphedema.
REFERENCES
1. International Society of Lymphology. The diagnosis and treatment of peripheral lymphedema: 2013 Consensus Document of the International Society of Lymphology. Lymphology. 2013;46(1):1- 11.
2. Morgan PA, Franks PJ, Moffatt CJ. Healthrelated quality of life with lymphoedema: a review of the literature. Int Wound J. 2005;2(1):47-62.
3. Mortimer PS, Rockson SG. New developments in clinical aspects of lymphatic disease. J Clin Invest. 2014;124(3):915-921.
4. Levick JR, Michel CC. Microvascular fluid exchange and the revised Starling principle. Cardiovasc Res. 2010;87(2):198-210.
5. Kinmonth JB, Taylor GW, Tracy GD, Marsh JD. Primary lymphedema; clinical and lymphangiographic studies in a series of 107 patients in which the lower limbs were affected. Br J Surg. 1957;45(189):1-9.
6. Saito Y, Nakagami H, Kaneda Y, Morishita R. Lymphedema and therapeutic lymphangiogenesis. Biomed Res Int. 2013;2013:804675.
7. Cormier JN, Askew RL, Mungovan KS, Xing Y, Ross MI, Armer JM. Lymphedema beyond breast cancer: a systematic review and meta-analysis of cancerrelated secondary lymphedema. Cancer. 2010;116(22):5138-5149.
8. Carlson JA. Lymphedema and subclinical lymphostasis (microlymphedema) facilitate cutaneous infection, inflammatory dermatoses, and neoplasia: a locus minoris resistentiae. Clin Dermatol. 2014;32(5):599-615.
9. Campisi C, Boccardo F, et al. Microsurgical techniques for lymphedema treatment: derivative lymphatic-venous microsurgery. World J Surg. 2004;28(6):609-13.
10. Damstra RJ, Voesten HG, Klinkert P, Brorson H. Circumferential suctionassisted lipectomy for lymphoedema after surgery for breast cancer. Br J Surg. 2009;96(8):859-864.
11. Devoogdt N, Lemkens H, Geraerts I, et al. A new device to measure upper limb circumferences: validity and reliability. Int Angiol. 2010;29(5):401-407.
12. Hidding JT, Viehoff PB, Beurskens CH, van Laarhoven HW, Nijhuis-van der Sanden MW, van der Wees PJ. Measurement properties of instruments for measuring of lymphedema: systematic review. Phys Ther. 2016;96(12):1965- 1981.
13. Stanton AW, Northfield JW, Holroyd B, Mortimer PS, Levick JR. Validation of an optoelectronic limb volumeter (Perometer). Lymphology. 1997;30(2):77- 97.
14. Gebruers N, Truijen S, Engelborghs S, De Deyn PP. Volumetric evaluation of upper extremities in 250 healthy persons. Clin Physiol Funct Imaging. 2007;27(1):17-22.
15. Damstra RJ, Glazenburg EJ, Hop WC. Validation of the inverse water volumetry method: a new gold standard for arm volume measurements. Breast Cancer Res Treat. 2006;99(3):267-273.
16. Moseley A, Piller N. Reliability of bioimpedance spectroscopy and tonometry after breast conserving cancer treatment. Lymphat Res Biol. 2008;6(2):85-87.
17. Shah C, Arthur DW, Wazer D, Khan A, Ridner S, Vicini F. The impact of early detection and intervention of breast cancer-related lymphedema: a systematic review. Cancer Med. 2016;5(6):1154- 1162.
18. Partsch H, Stöberl C, Urbanek A, Wenzel-Hora BI. Clinical use of indirect lymphography in different forms of leg edema. Lymphology. 1988;21(3):152- 160.
19. Itkin M. Lymphatic intervention in a new frontier of IR. J Vasc Interv Radiol. 2014;25(9):1404-1405.
20. Devoogdt N, Pans S, De Groef A, et al. Postoperative evolution of thickness and echogenicity of cutis and subcutis of patients with and without breast cancerrelated lymphedema. Lymph Res Biol. 2014;12(1):23-31.
21. Suehiro K, Morikage N, Murakima M, et al. Subcutaneous tissue ultrasonography in legs with dependent edema and secondary lymphedema. Ann Vasc Dis. 2014;7(1):21-27.
22. Naouri M, Samimi M, Atlan M, et al. High-resolution cutaneous ultrasonography to differentiate lipoedema from lymphoedema. Br J Dermatol. 2010;163(2):296-301.
23. Lee BB, Andrade M, Antignani PL, et al. Diagnosis and treatment of primary lymphedema. Consensus document of the International Union of Phlebology (UIP)-2013. Int Angiol. 2013;32(6):541- 574.
24. O’Donnell TF Jr, Rasmussen JC, Sevick- Muraca EM. New diagnostic modalities in the evaluation of lymphedema. J Vasc Surg Venous Lymphat Disord. 2017;5(2):261-273.
25. White RD, Weir-McCall JR, Budak MJ, Waugh SA, Munnoch DA, Sudarshan TA. Contrast-enhanced magnetic resonance lymphography in the assessment of lower limb lymphoedema. Clin Radiol. 2014;69(11):e435-e444.
26. Sherman AI, Ter-Pogossian M. Lymphnode concentration of radioactive colloidal gold following interstitial injection. Cancer. 1953;6(6):1238-1240.
27. Henze E, Schelbert HR, Collins JD, Najafi A, Barrio JR, Bennett LR. Lymphoscintigraphy with Tc-99m-labeled dextran. J Nucl Med. 1982;23(10):923- 929.
28. Szuba A, Shin WS, Strauss HW, Rockson S. The third circulation: radionuclide lymphoscintigraphy in the evaluation of lymphedema. J Nucl Med. 2003;44(1):43-57.
29. Nguyen AT, Suami H, Hanasono MM, Womack VA, Wong FC, Chang EI. Longterm outcomes of the minimally invasive free vascularized omental lymphatic flap for the treatment of lymphedema. J Surg Oncol. 2017;115(1):84-89.
30. Modi S, Stanton AW, Mortimer PS, Levick JR. Clinical assessment of human lymph flow using removal rate constants of interstitial macromolecules: a critical review of lymphoscintigraphy. Lymphat Res Biol. 2007;5(3):183-202.
31. Aldrich MB, Guilliod R, Fife CE, et al. Lymphatic abnormalities in the normal contralateral arms of subjects with breast cancer-related lymphedema as assessed by near-infrared fluorescent imaging. Biomed Opt Express. 2012;3(6):1256- 1265.
32. Alander J, Kaartinen I, Laakso A, et al. A review of indocyanine green fluorescent imaging in surgery. Int J Biomed Imaging. 2012;2012:940585.
33. Zhu B, Sevick-Muraca EM. A review of performance of near-infrared fluorescence imaging devices used in clinical studies. Br J Radiol. 2015;88(1045):20140547.
34. Chi C, Du Y, Ye J, et al. Intraoperative imaging-guided cancer surgery: from current fluorescence molecular imaging methods to future multi-modality imaging technology. Theranostics. 2014;4(11):1072-84.
35. Marshall MV, Rasmussen JC, Tan IC, et al. Near-infrared fluorescence imaging in humans with indocyanine green: a review and update. Open Surg Oncol J. 2010;2(2):12-25.
36. Rasmussen JC, Tan IC, Marshall MV, et al. Human lymphatic architecture and dynamic transport imaged using near-infrared fluorescence. Transl Oncol. 2010;3(6):362-372.
37. Tan IC, Maus EA, Rasmussen JC, et al. Assessment of lymphatic contractile function after manual lymphatic drainage using near-infrared fluorescence imaging. Arch Phys Med Rehabil. 2011;92(5):756- 764.e1.
38. Unno N, Inuzuka K, Suzuki M, et al. Preliminary experience with a novel fluorescence lymphography using indocyanine green in patients with secondary lymphedema. J Vasc Surg. 2007;45(5):1016-1021.
39. Suzuki M, Unno N, Yamamoto N, et al. Impaired lymphatic function recovered after great saphenous vein stripping in patients with varicose vein: venodynamic and lymphodynamic results. J Vasc Surg. 2009;50(5):1085-1091.
40. Yamamoto T, Matsuda N, Doi K, et al. The earliest finding of indocyanine green lymphography in asymptomatic limbs of lower extremity lymphedema patients secondary to cancer treatment: the modified dermal backflow stage and concept of subclinical lymphedema. Plast Reconstr Surg 2011;128(4):314e-321e.
41. Yamamoto T, Narushima M, Doi K, et al. Characteristic indocyanine green lymphography findings in lower extremity lymphedema: the generation of a novel lymphedema severity staging system using dermal backflow patterns. Plast Reconstr Surg. 2011;127(5):1979-1986.
42. Yamamoto T, Yamamoto N, Doi K, et al. Indocyanine green-enhanced lymphography for upper extremity lymphedema: a novel severity staging system using dermal backflow patterns. Plast Reconstr Surg. 2011;128(4):941-947.
43. Yamamoto T, Narushima M, Yoshimatsu H, et al. Indocyanine green velocity: lymph transportation capacity deterioration with progression of lymphedema. Ann Plast Surg. 2013;71(5):591-594.
44. Yamamoto T, Narushima M, Yoshimatsu H, et al. Dynamic indocyanine green (ICG) lymphography for breast cancerrelated arm lymphedema. nn Plast Surg. 2014;73(6):706-709.
45. Belgrado JP, Vandermeeren L, Vankerckhove S, et al. Near-infrared fluorescence lymphatic imaging to reconsider occlusion pressure of superficial lymphatic collectors in upper extremities of healthy volunteers. Lymphat Res Biol. 2016;14(2):70-77.
46. Mihara M, Hara H, Araki J, et al. Indocyanine green (ICG) lymphography is superior to lymphoscintigraphy for diagnostic imaging of early lymphedema of the upper limbs. PLoS One. 2012;7(6):e38182.
47. Akita S, Mitsukawa N, Kazama T, et al. Comparison of lymphoscintigraphy and indocyanine green lymphography for the diagnosis of extremity lymphoedema. J Plast Reconst Aesthet Surg. 2013;66(6):792-798.